New Direct Air Capture Center will leverage Georgia Tech’s leadership in a burgeoning field.
(text and background only visible when logged in)
In 2015, nearly 200 countries agreed: they would reduce their emissions of carbon dioxide and other greenhouse gases to limit warming of the earth’s atmosphere to well below 2 degrees Celsius.
The Paris Agreement actually aims for 1.5 degrees above pre-industrial levels to avoid potential catastrophic changes to our climate. But it’s become increasingly clear to climate scientists and policymakers that just reducing emissions is not enough.
“We now know that we probably should have stopped putting massive amounts of CO2 in the air 10, 20, 30 years ago to prevent the climate from getting above 2 degrees C,” said Chris Jones, a chemical engineer at Georgia Tech. “Now we've waited so long to reduce our emissions that we need to develop technologies that are referred to as negative emissions technologies that remove CO2 from the atmosphere.”
Jones was one of a handful of scientists who co-authored a landmark National Academies report in 2018 that outlined a variety of approaches to negative emissions. Agricultural practices and forest management are options — essentially using nature’s ability to grab carbon dioxide out of the air and lock it away in plants and soil. But Jones said we’ll need quicker and more direct approaches.
“We could plant billions of trees to do this, but there's not enough available land. And the trees don't grow fast enough for us to do this quickly enough to slow global warming at the rate required,” said Jones, John F. Brock III School Chair in the School of Chemical and Biomolecular Engineering (ChBE). “That's where direct air capture comes in: It's a chemical engineering way of designing a process that takes CO2 out of the air.”
Direct air capture is a bit like a massive household air purifier — but for the globe. Systems would pull air across specially designed filter materials with molecules that grab CO2. When the filters are saturated, they’re cleaned, and the carbon dioxide is pumped underground for storage in the very places we’ve extracted oil and natural gas over the decades.
It’s a technology proposed only in 1999, with companies launched in 2008, and is now quickly becoming a reality, according to another Georgia Tech chemical engineer, Matthew Realff.
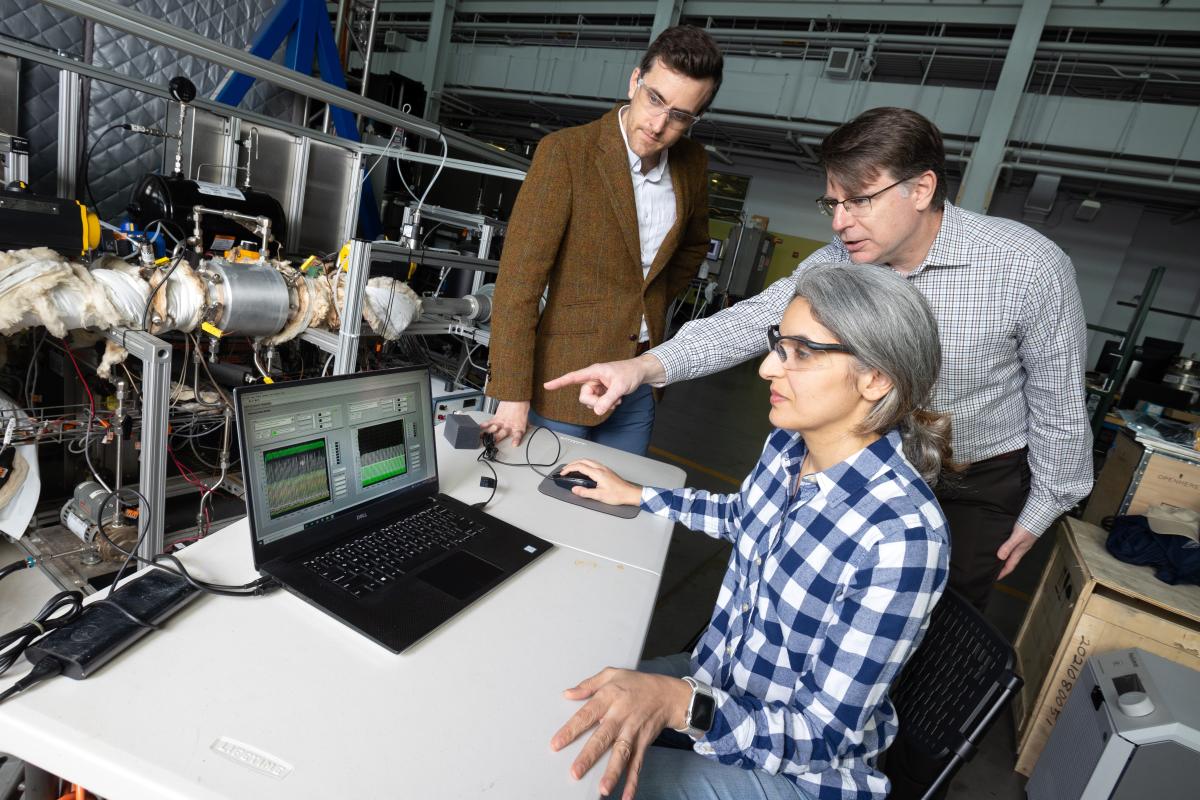
Postdoctoral fellow Poorandokht Kashkouli, seated, discusses test data from their direct air capture rig with Ryan Lively, left, and Chris Jones. The system pulls air across filter materials to remove carbon dioxide.
“It's definitely a technology that is moving past the lab; it's in the pilot scale/deployment phase as an initial technology. By 2030, we should see deployment of what I would call the first commercial-scale facilities in different places in the United States — systems that can remove a million tons of CO2 a year,” said Realff, professor and David Wang Sr. Fellow in ChBE. He pointed to a $3.5 billion federal investment to develop four regional direct air capture hubs that was part of federal infrastructure legislation passed in 2021.
“If you're going to make a difference, to be honest, it really needs to be at about 1,000 times that scale, a gigaton scale of direct air capture,” Realff continued. “Some people would argue that we might need even more than that two to three decades from now, depending on how our emissions reduction efforts go.”
Realff and Jones are working at different ends of the direct air capture spectrum — the systems and molecular levels — to develop the technology. In between is ChBE professor Ryan Lively, who works on materials, devices, and processes. Now they’re recruiting more of their Georgia Tech colleagues to the cause with a newly established Direct Air Capture Center (DirACC) within Tech’s Strategic Energy Institute.
The center is an effort to seed interesting ideas across engineering, sciences, policy, and more and leverage Georgia Tech’s longstanding leadership in the area. Lively said DirACC will fill a need nationally to act as a convener of researchers, industry, funders, and other stakeholders. It is one of a few centers in the nation focused on direct air capture and the first such effort to encompass the complete supply chain of capture and sequestration of CO2 from the air.
(text and background only visible when logged in)
By 2030, we should see deployment of what I would call the first commercial-scale facilities in different places in the United States — systems that can remove a million tons of CO2 a year.
MATTHEW REALFF
(text and background only visible when logged in)
Leveraging Longstanding Leadership
“As an institution, Georgia Tech has essentially been involved since the direct air capture field’s infancy,” Jones said.
Jones has led collaborations since 2008 with one of the original startup companies in the field, Global Thermostat. Lively leads an Energy Frontier Research Center that received a rare third round of funding from the U.S. Department of Energy in 2022 and that’s working on how materials for clean-energy technology evolve and degrade. One of the focus areas is direct air capture.
Alongside the 2018 National Academies report, Congress introduced a federal tax credit for removing carbon dioxide from the air. Lawmakers more than tripled those incentives to $180 per ton in the 2022 Inflation Reduction Act. Jones said that got people’s attention.
“Since 2018, we've had billions of dollars of legislation for direct air capture technology research and development. That trend is why we are launching the Direct Air Capture Center now: We really want to communicate to the outside that we are a hub in this space.”
Tim Lieuwen, executive director of Georgia Tech’s Strategic Energy Institute, said the new center will extend beyond developing the science and technology: “It’s exciting to see Georgia Tech also engaging more broadly in the societal-level considerations associated with deployment of direct air capture, including community engagement, workforce development, and ensuring a just transition for historically marginalized populations.”
(text and background only visible when logged in)
Just Like in Real Estate, Location Matters
Jones works at the first step of the carbon-capture process: the molecules that link with CO2 to pull it out of the air. One of the areas he’s become most interested in is customizing different materials for different locations and climates.
“A molecule or a material that we studied five years ago maybe failed operating at 80 degrees Fahrenheit. That would not work in Atlanta or in Florida, but maybe it works really well if we go to 50 degrees F or 20 degrees F, and we can deploy it in Montana,” Jones said. “We're starting to think more about whether we might have advantaged materials or advantaged processes in particular locations.”
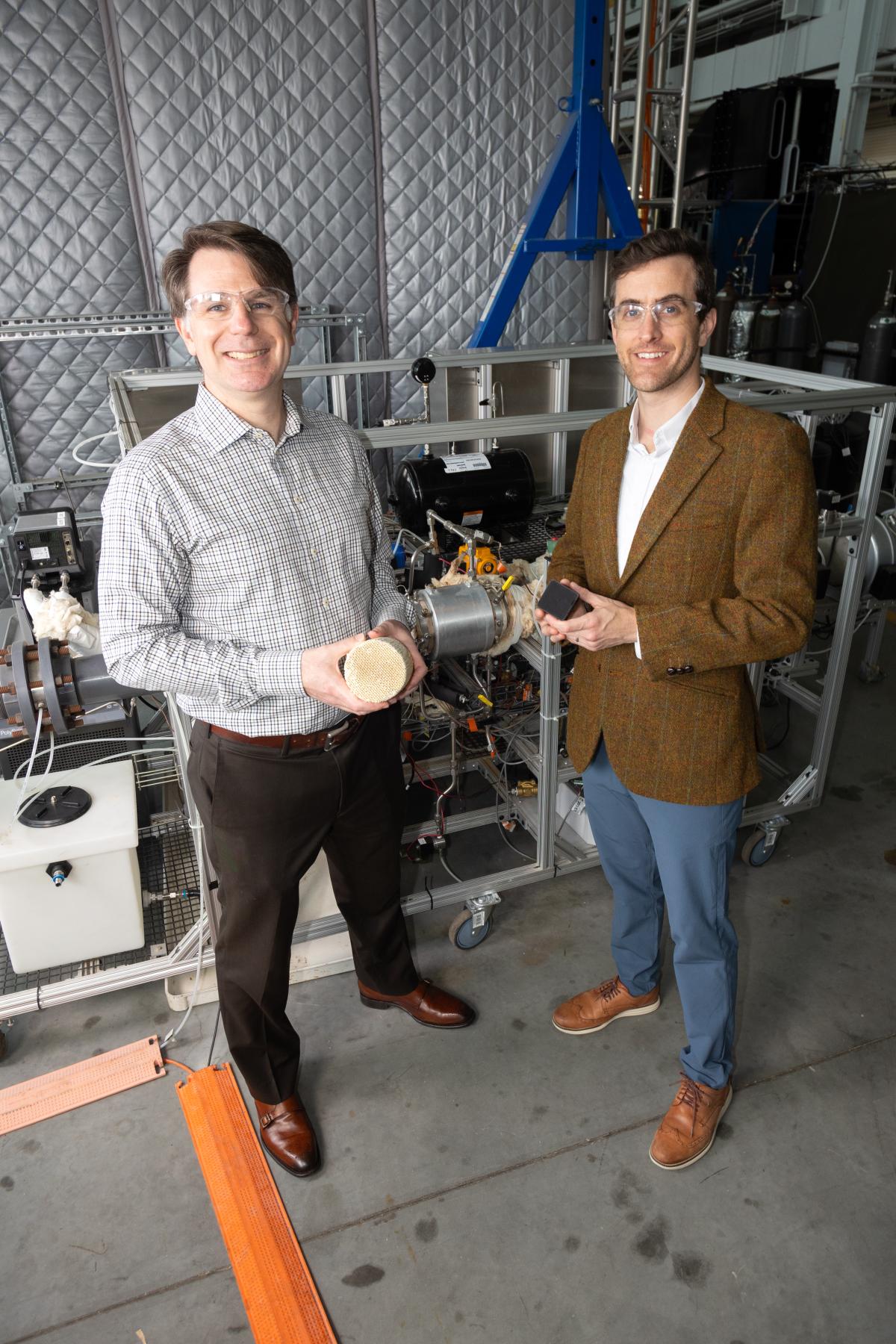
Chris Jones, left, and Ryan Lively hold two kinds of filters for direct air capture systems. The test rig behind them uses the larger filter Jones is holding.
In a future where direct air capture is widely deployed — say, 20 years from now — Jones said there could be a dozen different solutions customized to different locations around the globe. He said Georgia Tech has an opportunity to get ahead of that curve and design the right solutions for the right environments.
Realff’s work on systems and process modeling plugs in here. His team works to extrapolate the economics and the lifecycle of materials and lab-scale direct air capture modules to real-world performance. If Jones and Lively have data from experiments at different temperatures and humidities, for example, Realff might model how those materials would work over the course of a year.
“That's the frontier of where we're working right now, understanding how environmental variability impacts the performance of the direct air capture system,” Realff said.
Practicality Matters, Too
Another frontier is engineering carbon capture materials that will last long enough to be economically practical, Lively said.
When Jones creates molecules that grab carbon dioxide out of the air, Lively incorporates them into fibers that can be bundled together or even woven into fabric. Those fibers seem to be durable, but the question is whether the delicate chemistry of the molecules repeatedly capturing and then releasing the CO2 can hold up. The DOE-funded center Lively leads is working in part to better understand how these materials evolve and degrade.
“One of the key cost drivers is, how long can you make these things last?” Lively said. “We know what the answer should be: If you can get them to where they largely maintain their performance for a year to a year and a half, then you're in good shape. We're just not sure if we can get there yet.”
Creating fibers for use in a canister, filter, or other device has become a fairly mature technology, Lively said, so the team is working now to move some of their materials into the commercial market. He’s also experimenting with 3D printing approaches that can create more complex structures with the materials — though at a cost.
“The nice part about 3D printing is you can get really complex structures that you can't get with fibers. But the manufacturing process is fundamentally slower,” Lively said. “The complexity of the device is going to have to make up for the slower rate at which you can make it, because the slower rate will mean higher cost.”
(text and background only visible when logged in)
Air Capture and Renewables as a One-Two Punch
One of the newer projects Realff, Jones, and Lively are working on would pair carbon capture systems with wind farms.
They’re exploring systems that would be passive: Instead of relying on some mechanism to blow or draw air across the filters that extract CO2, natural wind would move air across the filters in these systems. It turns out, a wind speed of around 6 meters per second is enough velocity, Realff said — and it’s also just enough to turn a wind turbine and generate power, although higher velocities are preferred.
“If you integrate passive capture with wind farms, now your turbines are helping mix the air. You could think about having alternating banks of direct air capture and then turbines,” Realff said. “The turbines generate the power to enable the direct air capture system to work; you don't have to connect either one of them to the grid.”
Realff said the system the researchers are developing is relatively simple, using the wind electricity to heat up a resistor and release the carbon dioxide and regenerate the filters so they can remove more CO2. Lively called this “all-electric” approach a second-generation system. Current direct air capture technology uses steam or hot water, for example, to heat the filters and release the captured CO2.
“If you think about it, I want to put a passive contactor where I have a lot of wind — which is also where I want to build a wind farm. There’s a natural coupling of those two,” Realff said.
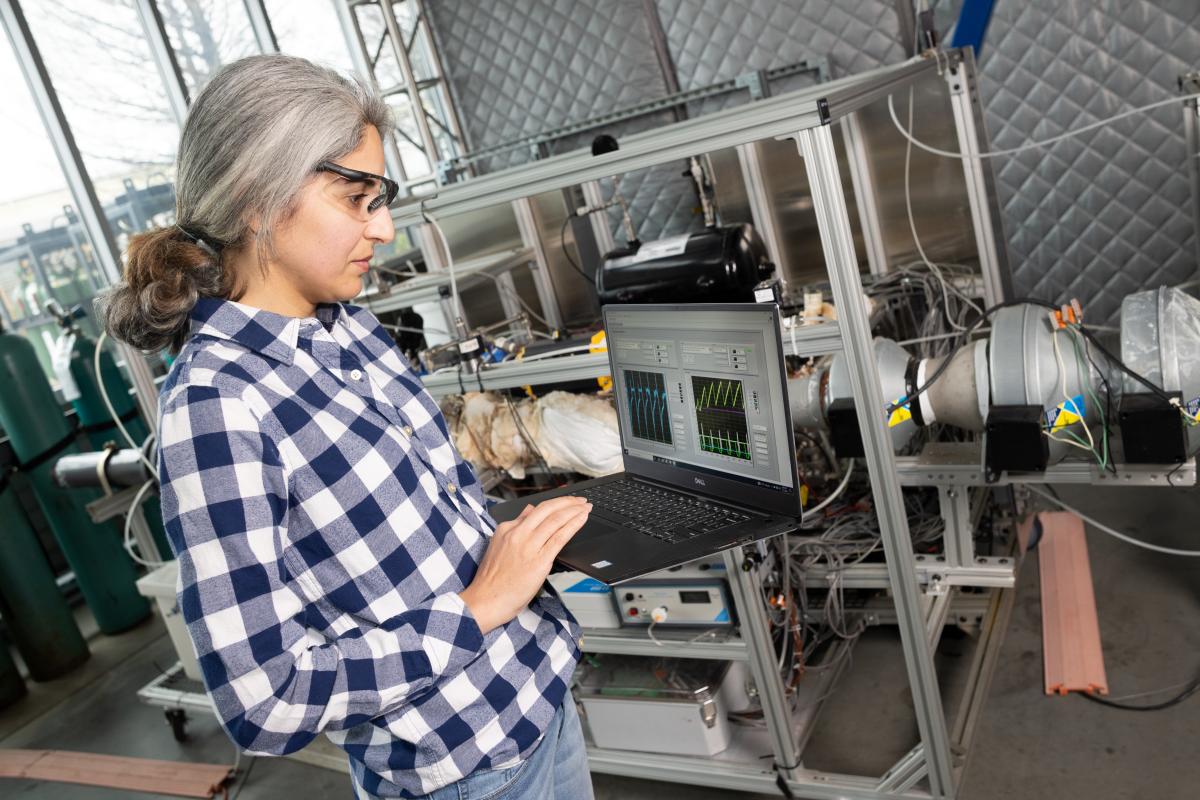
Postdoctoral fellow Poorandokht Kashkouli with the direct air capture rig in Georgia Tech's Carbon Neutral Energy Solutions Laboratory.
When A Double-Negative is a Positive
Another emerging idea would pair direct air capture systems with natural gas combined-cycle power plants. These plants use gas to turn a turbine and generate electricity. The waste heat from that process is then used to create steam that turns another turbine and generates more power.
Realff, Jones, and Lively have partnered with ChBE’s Fani Boukouvala and Joe Scott on a project funded by the Advanced Research Projects Agency-Energy to instead use that steam to do conventional post-combustion carbon capture and to power a direct air capture system.
“With the combination of those two systems, we can get natural gas combined-cycle plants that can operate and be net negative in their carbon dioxide,” Realff said.
In fact, he said, the reduction in carbon from such a system would be enough to wipe out the carbon emissions of another power plant operating at full capacity.
“For power companies, it gives you a way to get to essentially zero carbon by modifying, say, roughly half your fleet of natural gas combined-cycle plants.”
No Time to Waste
Those kinds of advances will be critical to making direct air capture economical enough to have impact, the researchers said.
Jones called it a generational challenge akin the NASA’s failure-is-not-an-option mantra during the 1960s moon missions.
“The level of funding and interest is pretty enormous, but the challenge, unfortunately, might be even more enormous than the interest,” Lively said. “The longer we wait, the bigger the challenge.”
(text and background only visible when logged in)
Solar energy technology was promising but too expensive to be practical when it debuted in the late 1970s and early ‘80s. Now, it’s cheap and common. The difference with direct air capture is the timeline: We can’t afford to wait 40 years to scale.
Jones pointed to solar energy as a useful analogy for getting to a future with widespread removal of atmospheric carbon. Initially, the technology was promising but too expensive to be practical when it debuted in the late 1970s and early ‘80s. Now, it’s cheap and common. The difference with direct air capture is the timeline: We can’t afford to wait 40 years to scale.
“Today, direct air capture from the initial startup companies costs between, say, $600 and $1,000 a ton. The target, in order to have it be widespread deployed all over the world, is about $100 a ton,” he said. “We can't have a five- or 10-year technology testing period; it's got to be a year or 18 months so that we can build it, operate it, learn from it, revise it, build it, operate it, learn from it. We need to do that five or 10 times to get the cost down.”
Related Content
DOE Renews Funding of Energy Frontier Research Center with $13.2 Million Grant
The center receives a third round of funding for the study of materials used in clean energy technologies.