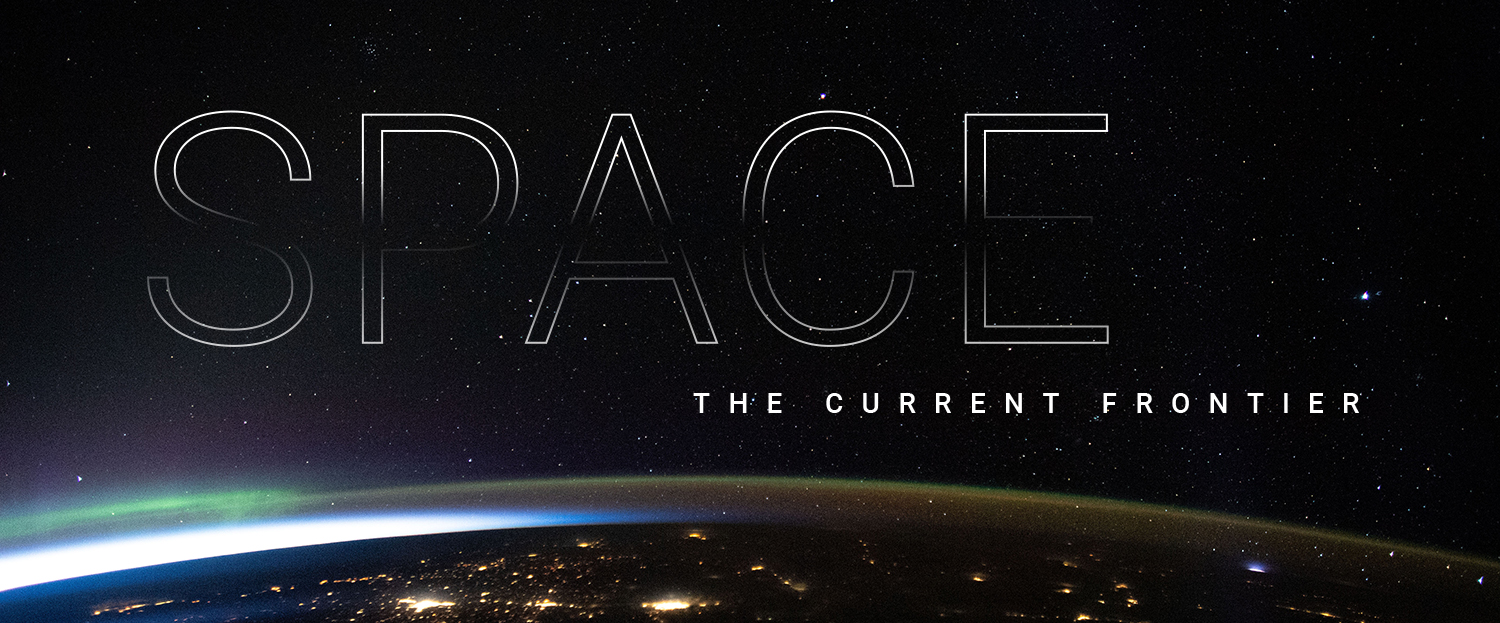
(text and background only visible when logged in)
Our engineers have the solar system covered, with projects hundreds — or millions — of miles from home.
(text and background only visible when logged in)
Right now, about 70 million miles away, a Ramblin’ Wreck from Georgia Tech streaks through the cosmos. It’s a briefcase-sized spacecraft called Lunar Flashlight that was assembled in a Georgia Tech Research Institute (GTRI) cleanroom in 2021, then launched aboard a SpaceX rocket in 2022.
The plan was to send Lunar Flashlight to the moon, where the spacecraft would shoot lasers at its south pole in a search for frozen water. Mission control for the flight was on Georgia Tech’s campus, where students in the Daniel Guggenheim School of Aerospace Engineering (AE) sat in the figurative driver’s seat. They worked for several months in 2023 to coax the craft toward its intended orbit in coordination with NASA’s Jet Propulsion Lab (JPL).
A faulty propulsion system kept the CubeSat from reaching its goal. Disappointing, to be sure, but it opened a new series of opportunities for the student controllers. When it was clear Lunar Flashlight wouldn’t reach the moon and instead settle into an orbit of the sun, JPL turned over ownership to Georgia Tech. It’s now the only higher education institution that has controlled an interplanetary spacecraft.
Lunar Flashlight’s initial orbit, planned destination, and current whereabouts mirrors much of the College of Engineering’s research in space technology. Some faculty are focused on projects in low earth orbit (LEO). Others have an eye on the moon. A third group is looking well beyond our small area of the solar system.
No matter the distance, though, each of these Georgia Tech engineers is working toward a new era of exploration and scientific discovery.

“Low earth” is the area that extends about 1,200 miles above our planet’s surface. To avoid falling back toward the ground, anything in LEO must circle Earth at about 17,000 miles an hour. The majority of satellites and the International Space Station are in this zone.
Georgia Tech’s LEO contributions stretch back to 1984, when the Space Shuttle Challenger ferried a 30-foot spacecraft — NASA’s Long Duration Exposure Facility — into orbit, where it remained for seven years. On board was an experiment led by GTRI’s Don Blue that studied how space’s harsh environment affects materials and opto-electronic components.
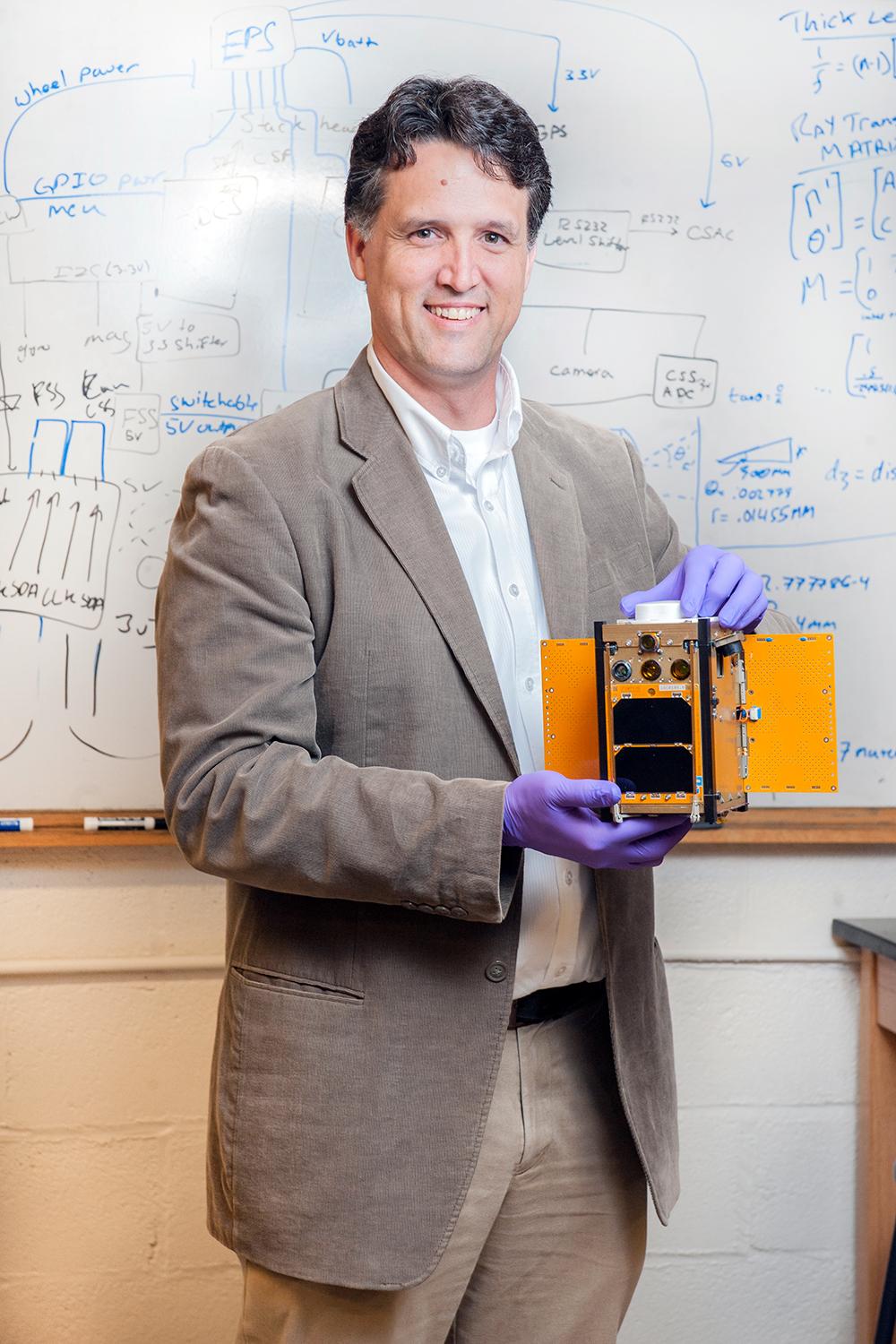
Brian Gunter with one of the RANGE satellites. (Photo: Christopher Moore)
In 1998, GTRI designed a radio antenna for the still-under-construction International Space Station. It was installed to provide communications to astronauts waiting in air locks prior to spacewalks.
Fifteen years later, Jud Ready — from GTRI and the School of Materials Science and Engineering — created carbon nanotube arrays that went to space aboard a nanosatellite called ALICE.
Things began to accelerate around that time with the arrival of a pair of AE School faculty members. Brian Gunter joined in 2013, bringing expertise in orbits, satellites, and more. Glenn Lightsey came two years later from the University of Texas at Austin, where he had built and flown several CubeSats. The small satellites were quickly gaining popularity in the aerospace community because of their small size and low cost.
Gunter soon began working on the Ranging and Nanosatellite Guidance Experiment (RANGE) mission, a pair of satellites designed to follow each other in orbit with a goal of improving positioning capabilities of small nanosatellites, which have a mass of 10 kilograms or less. RANGE launched in December 2018, becoming Georgia Tech’s first small satellite to reach orbit.
Another CubeSat designed, fabricated, and tested on campus caught a lift to space a few months later. Prox-1 rode aboard a SpaceX Falcon Heavy in June 2019. The project, co-led by Lightsey and former Professor of the Practice David Spencer, was two satellites in one: It carried and deployed LightSail, a solar sail demonstration mission built by the California Polytechnic State University. Both spacecraft continue to circle the Earth six years after their deployment.
Seven more launches carrying Georgia Tech projects flew in the next three years as the College’s researchers built a small spacecraft pipeline that continues today. Another dozen or so projects are expected to launch in the next three or four years.
Gunter attributed Georgia Tech’s accelerated presence in LEO to two developments. First is the increased access to space made possible by programs sponsored by NASA, the National Science Foundation (NSF), and the Department of Defense. Many of those programs specifically promote ridesharing for small satellites created by universities.
The second is the growth in skillsets and resources on campus. He and Lightsey are continually seeking out and investing in “smallsat” opportunities.
“These projects take a full range of skills: systems engineering, software development, structures, electrical systems, and flight operations,” said Gunter, associate professor and director of the Space Systems Design Lab. “It takes large teams of students with different backgrounds and years of investment. So, while the opportunities provided by NASA, NSF, and others are open and available to any university, it takes a certain level of determination to manage all aspects of a multiyear mission.”
Gunter’s lab currently includes nine faculty members and more than 90 graduate students — all focused on space-related research. That means they have the breadth and depth necessary to manage those complex undertakings. Lightsey said the future missions also are a testament to the past, capitalizing on investments Georgia Tech made earlier this century to create a small spacecraft flight program.
“It fits well with Tech’s emphasis on modern applied engineering,” said Lightsey, John W. Young Chair and professor. “Before Brian and I arrived, a few people, like Jud [Ready], were doing their own payloads and experiments on the Space Shuttle and International Space Station. We joined and became part of a critical mass of people working on spaceflight projects. We have continued to hire faculty members who work on flight projects, and that’s created a culture of spaceflight missions at Georgia Tech.
“Meanwhile, spacecraft have been getting smaller and less expensive, and launches occur more frequently,” Lightsey said. “All of this has made it possible for universities to have active flight programs.”
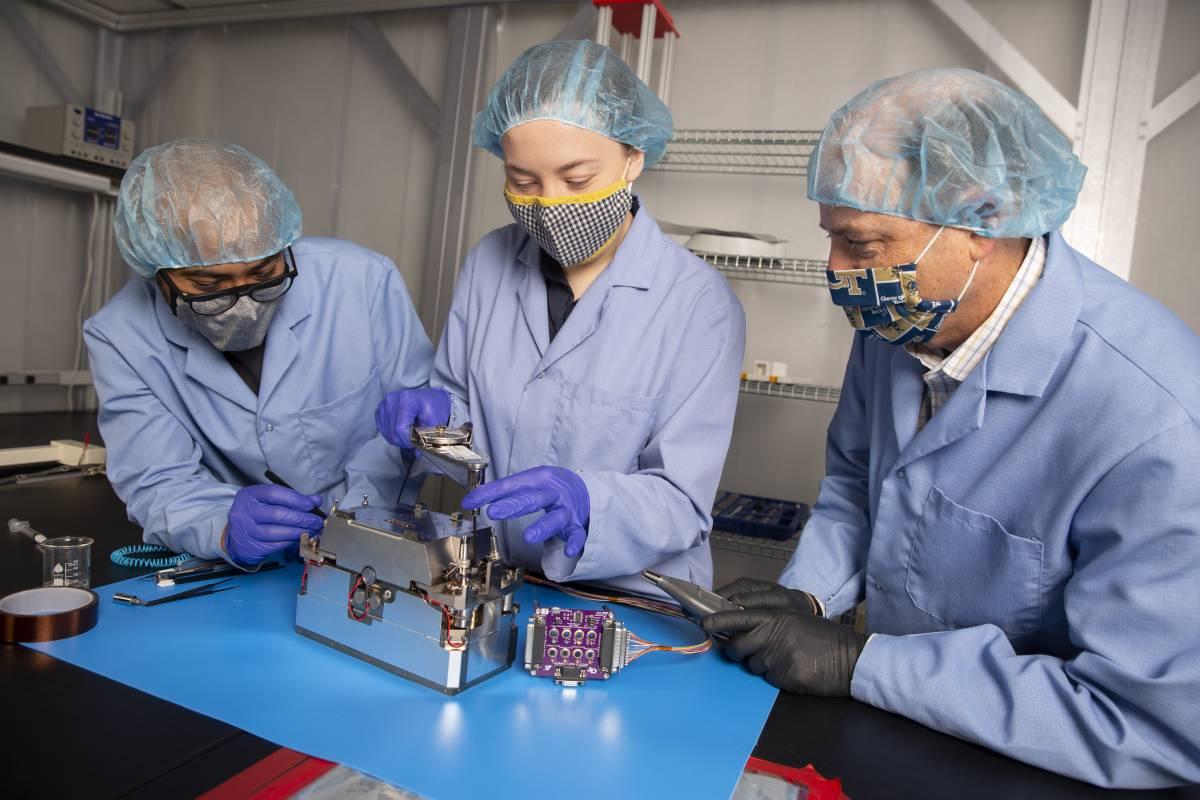
Glenn Lightsey (right) and students work on Lunar Flashlight’s propulsion system. (Photo: Candler Hobbs)
The next set of projects bound for orbit include more CubeSats built on campus and new kinds of propulsion systems to power them. For instance, Lightsey’s team designed and built the “prop” systems for six small spacecraft that will fly in formation as part of a NASA mission to study the sun. Another system will be constructed for a 2029 mission to test CubeSat technology that could someday study exoplanets.
Gunter’s upcoming flights include the creatively (and appropriately) named WEBS (Wireless Energy from Beamed Signals) mission. It’s funded by the Air Force Research Lab (AFRL) and the University Nanosatellite Program through a program that tasks researchers with finding better ways to use solar power in space.
The mission calls for AFRL’s Arachne satellite to gather energy from its solar panels, convert it to a radio frequency, then beam it to Gunter’s WEBS CubeSat hundreds of miles away, where it will be converted back to power. It would be the first demonstration of space-to-space solar power beaming.
Current spacecraft must independently generate and transport their own power. Beaming power across space would instead open the possibility of distributing power wherever it’s needed.
“We improve every time we finish a mission in low earth orbit, and this allows Georgia Tech to be more competitive for future opportunities,” Gunter said. “The first missions I led were spent focusing on basic subsystems, such as power and communications. Now that those are established, they can be carried forward into future projects and allow us to focus more on the technology being demonstrated. That experience and flight heritage is earned after many years, and it allows us to pursue increasingly challenging missions.”
(text and background only visible when logged in)
We improve every time we finish a mission in low earth orbit, and this allows Georgia Tech to be more competitive for future opportunities.
BRIAN GUNTER
(text and background only visible when logged in)

Only 12 people have walked on the moon, including Georgia Tech aerospace engineer John Young. In the past two years, however, Tech has returned to the lunar surface — this time with computer code instead of human feet.
Two algorithms created by Associate Professor John Christian and his students have helped guide two landers to the moon using optical navigation (OpNav) techniques Christian has been developing for more than a decade. OpNav uses cameras to help spacecraft know where they are in the solar system.
In February 2024, Christian’s algorithms helped Intuitive Machines’ Odysseus spacecraft achieve the first U.S. lunar landing since the Apollo program — and the first-ever successful landing by a commercial company. When Odysseus experienced an anomaly with its altimeter, the lander relied on a camera-based technique that was jointly developed by Christian and Intuitive Machines. The method tracked the apparent movement of landmarks from one image to another to help the lander understand its motion relative to the surface, allowing it to land and operate for 144 hours before entering standby mode.
A second Georgia Tech algorithm was onboard Intuitive Machines’ follow-up lander, Athena, this past March. It was largely the work of Ava Thrasher, a research engineer in the AE School who first got involved in OpNav research after taking Christian’s senior design course as an undergraduate.
She developed a crater detection algorithm that used image processing techniques to identify the centers of lunar craters. Then a second algorithm matched craters in the image to a catalog of known craters to help the spacecraft estimate its position and orientation. The goal was to create a reliable and quick onboard system to guide Athena to its landing site on Mons Mouton near the lunar south pole.
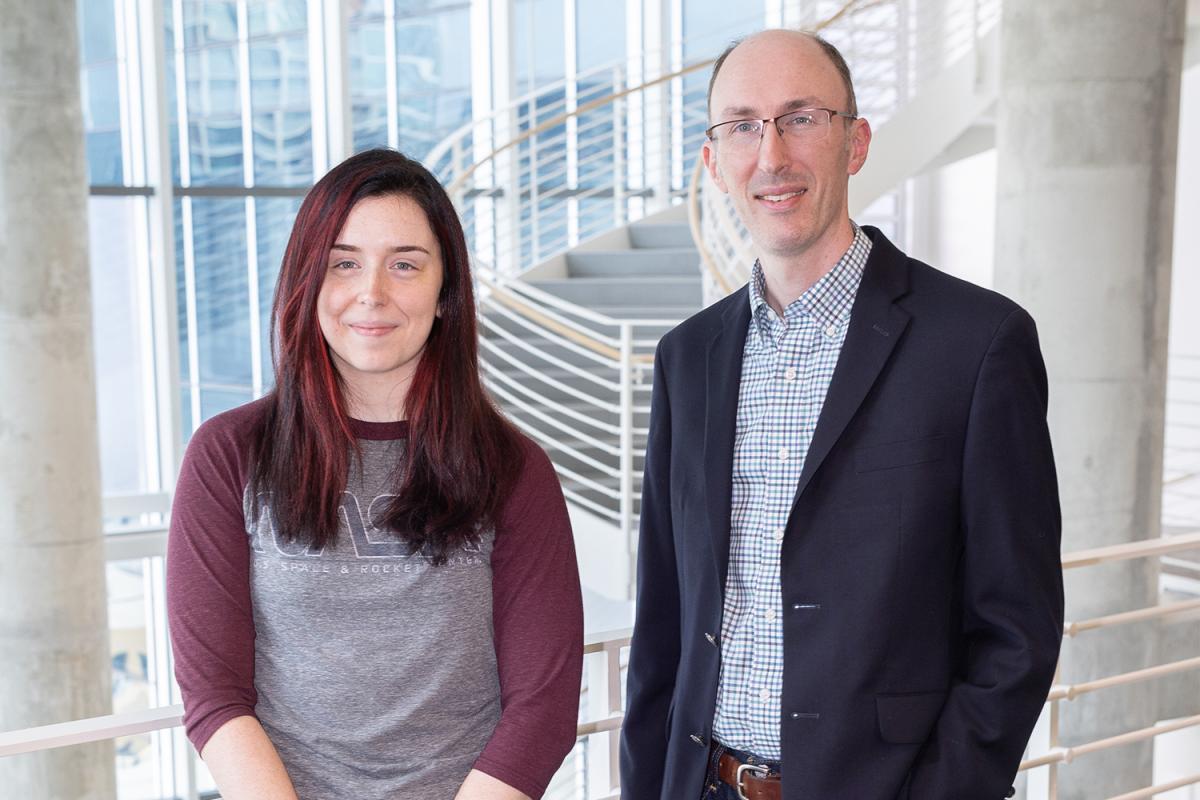
Ava Thrasher and John Christian’s algorithms, based on optical navigation research, have been used in recent lunar landing missions. (Photo: Candler Hobbs)
“These missions have given our students unique, hands-on learning experiences,” said Christian. “It’s been a privilege to provide some of the theoretical underpinnings that are helping to enable this new age of lunar exploration. Our collaborative partnerships with NASA, Intuitive Machines, and others are a perfect example of how academic research can be applied to open new frontiers.”
Intuitive Machine’s third lunar lander, expected to launch in December, will deliver an autonomous robotics algorithm developed by AE Associate Professor Yashwanth Nakka. Originally written during Nakka’s tenure at JPL, the software will allow a team of three different micro-rovers to cooperatively explore the lunar surface, conducting distributed science experiments without human supervision.
Past missions have mostly relied on a single rover to carry out a limited set of tasks. But deploying a team of robots — each with specialized instruments — unlocks a new operational paradigm.
YASHWANTH NAKKA
The mission forms the core of NASA’s Cooperative Autonomous Distributed Robotic Exploration (CADRE) initiative, which aims to demonstrate scalable autonomy for multiple robots in extreme planetary environments. CADRE is led by Jean-Pierre de la Croix, a three-time Georgia Tech graduate in electrical and computer engineering and computer science.
Nakka joined Georgia Tech in 2024 and is building a research laboratory designed to simulate lunar surface conditions. The lab includes lunar terrain that mimics mechanical interactions on the moon and the optical environment. The lab has engineered rock distributions, crater analogs, and lighting systems that simulate the harsh lighting and thermal gradients of lunar day-night cycles. The facility will support long-duration testing of autonomous teams of robots in varying terrain, lighting, and communication conditions.
“Past missions have mostly relied on a single rover to carry out a limited set of tasks. But deploying a team of robots — each with specialized instruments — unlocks a new operational paradigm,” Nakka explained. “Not only do we gain resilience by avoiding single points of failure, we also can enable dense, high-resolution data collection across multiple physical domains. This allows us to characterize the environment in a multi-modal, spatiotemporally coordinated way.”
Nakka’s work would help develop a major step toward autonomous robotic planetary exploration — where a team of robots adapts, cooperates, and dynamically reconfigures based on mission needs, the terrain they encounter, and the availability of energy. Nakka’s group also is developing new hardware, such as morphing rover wheels that can flex in granular terrain and stiffen on solid ground. Their variable tread height provides enhanced traction and energy efficiency across a variety of terrain.
“JPL is a phenomenal place for designing mission-ready technology,” Nakka said. “But Georgia Tech gives me the platform to tackle foundational research questions: How do you design and control robotic systems that are adaptable, resilient, and truly autonomous in unstructured, uncertain environments? These are the building blocks for a sustainable robotic presence on the moon, Mars, and beyond.”
(text and background only visible when logged in)
Other World Exploration

After earning his undergraduate physics degree from Georgia Tech in 1984, then going to grad school, John Cressler joined IBM to work on mixing silicon with germanium to create a new alloy for electronic chips. The team discovered that the combo was faster and more powerful than silicon alone. The technology revolutionized the electronics industry and now helps power GPS, WiFi, smartphones, and countless other modern devices.
Cressler continued his silicon-germanium (SiGe) research as a professor at Auburn University in the mid-1990s. One day, his Department of Defense program director asked if he had ever considered how SiGe would function in a radioactive environment. Cressler knew very little about the subject but agreed to explore it. It led to the world’s first SiGe radiation experiment.
“When nothing happened the first time, I asked my students if they did the experiment correctly,” said Cressler, Regents’ Professor and the Schlumberger Chair in Electronics in the School of Electrical and Computer Engineering (ECE). “We did it again, and nothing happened again. That’s when we learned SiGe is resistant to radiation.”
The discovery made Cressler a pioneer in the field. It also led him to space research. A current NASA project has him developing the electronics infrastructure for future missions to Europa, the most radioactive moon in the solar system.
Europa sits firmly in Jupiter’s magnetosphere and is constantly pelted with radiation. A typical medical X-ray delivers a radiation dose of .01 rem; a day on Europa would deliver 540 rem. Visiting Europa would kill a person, but it’s perfect for SiGe. It’s also considered the best place in the solar system to find life because it has a water ocean 10 kilometers below its surface.
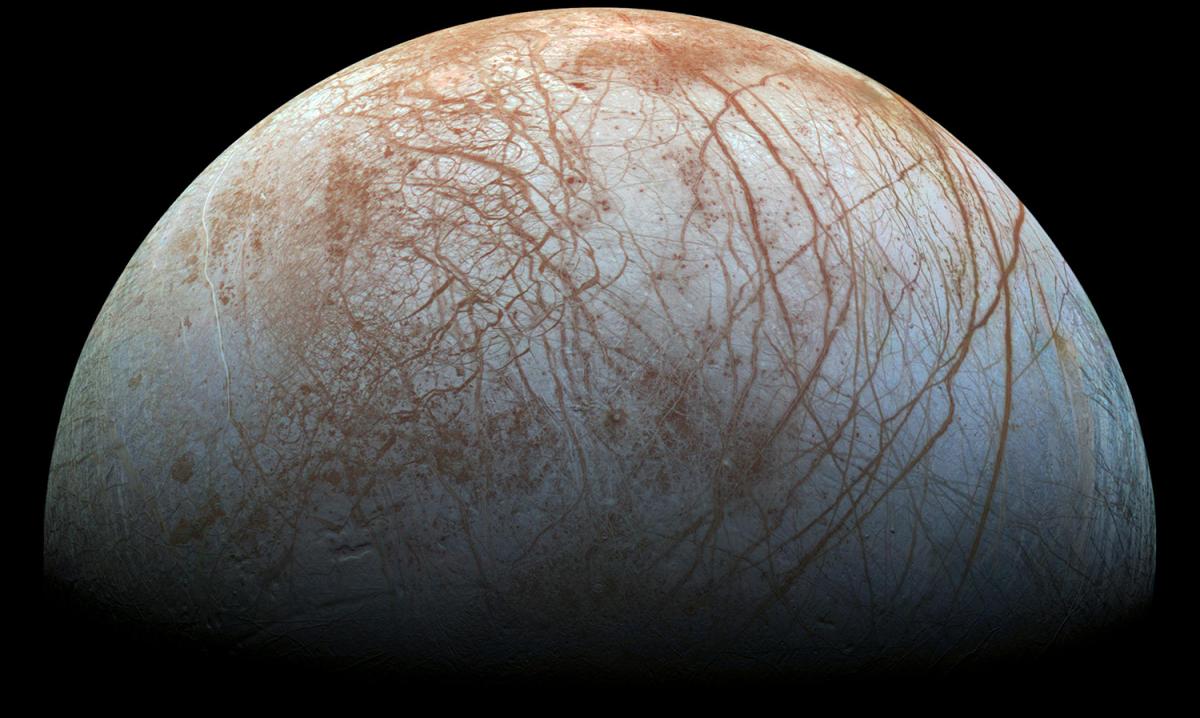
Long, linear cracks and ridges crisscross the surface ice of Europa. (Image courtesy: NASA)
Cressler and his students have already created an SiGe radio frequency receiver that successfully worked while being exposed to Europa-like conditions.
“This technology also is ideal for cold temperatures, which makes Europa even more ideal for SiGe transistors because its surface temperature is minus 300 degrees F,” said Cressler, who’s also talking to NASA about how the same kind of tech can be used for missions on Earth’s moon and its permanently shaded craters, which are even colder. “I was always interested in space as a kid, so working on a technology that is ideal for space has always jazzed me. There’s nothing cooler than thinking my stuff might enable humans to discover life someplace else someday.”
Whenever a rover arrives on Europa, the idea would be to drill through the surface ice until reaching water, then release a fleet of swimming robots to explore the ocean. That’s where Cressler’s ECE colleague Azadeh Ansari plays an important role. She specializes in building small robots equipped with sensors. She’s fabricated and tested sensors with JPL that would measure Europa’s water temperature, pressure, acidity, chemical composition, and more. Ansari’s chip would ride on JPL’s SWIM (Sensing With Independent Microswimmers) robots. It’s the first chip to combine so many sensors for ocean composition identification into a one-millimeter-sized package.
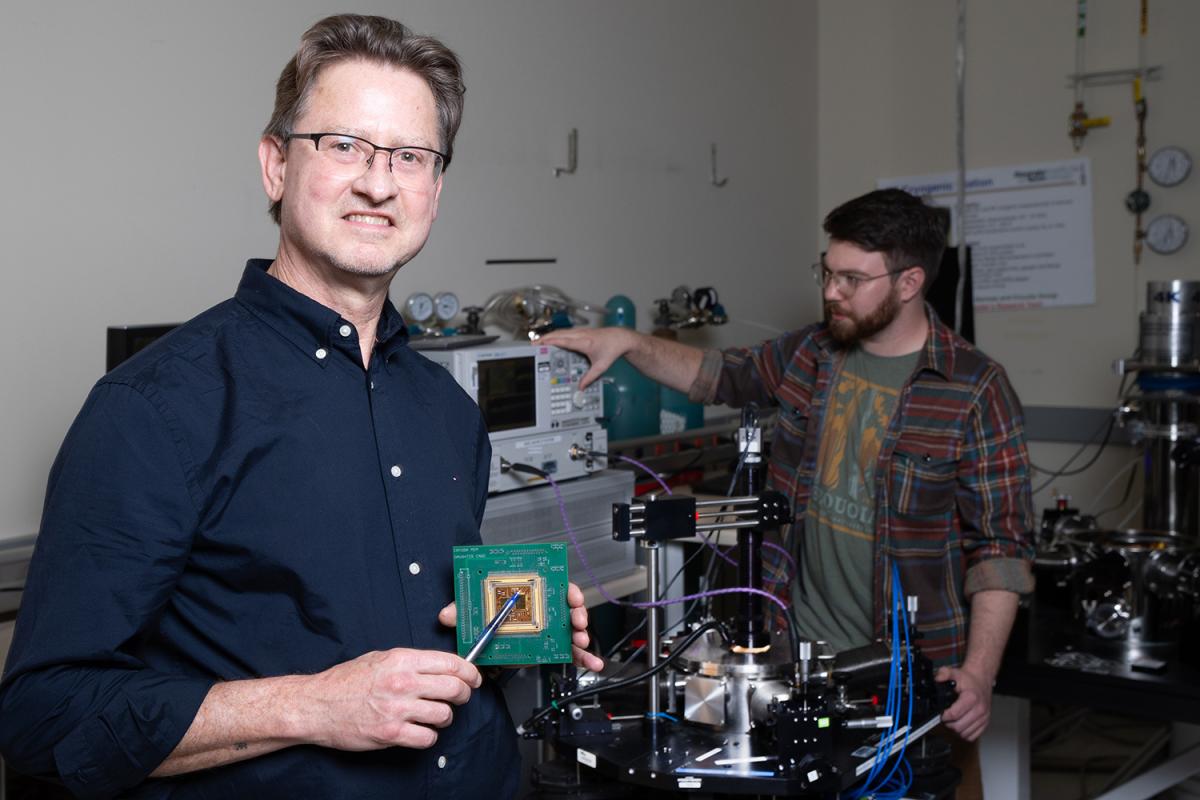
John Cressler’s discovery of silicon-germanium’s radiation resistance led him to space research. (Photo: Candler Hobbs)
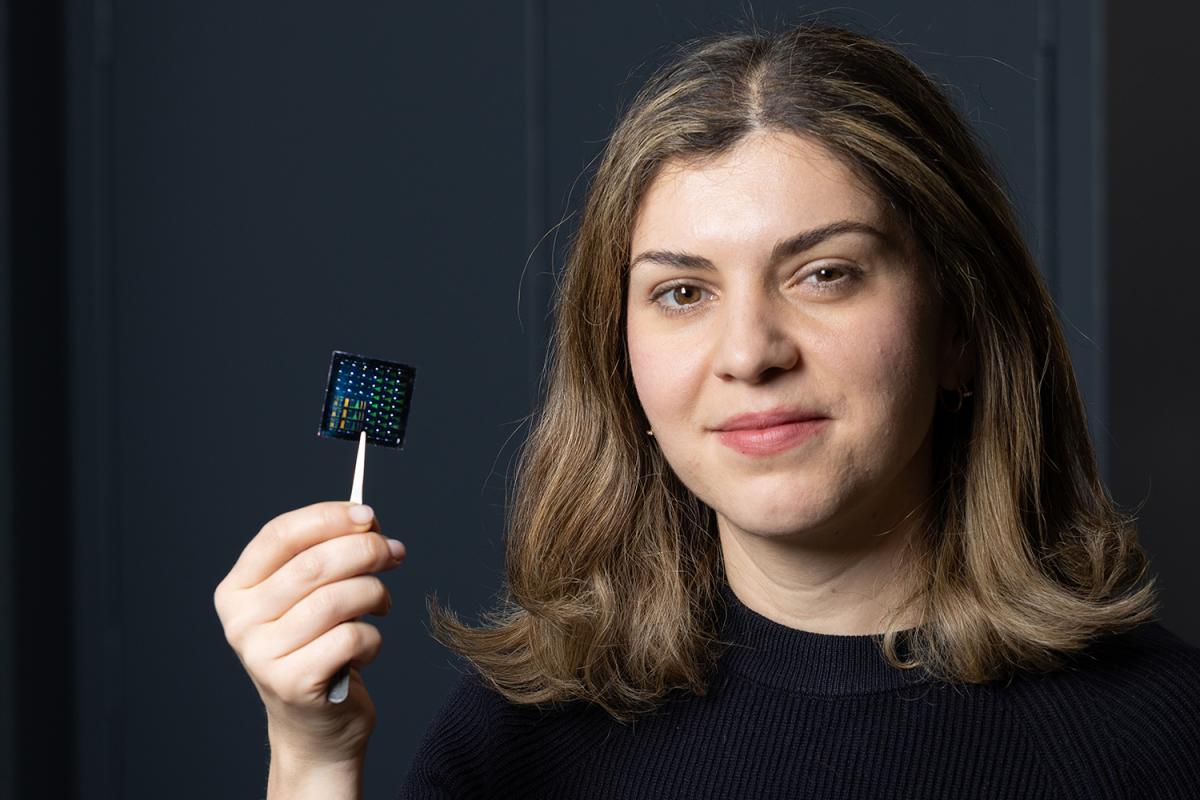
Azadeh Ansari has developed a chip that combines many sensors into one small package. (Photo: Candler Hobbs)
“Large robots are extremely limited in the water volume they can explore,” said Ansari, associate professor in ECE. “Having a team of small-scale robots with many sensors would allow us to explore and map a larger water volume more efficiently. The redundancy can also help overcome the fact that some machines will inevitably fail during the mission.”
Ansari’s next task is packaging the sensors as they prepare for mysterious ocean conditions. Scientists don’t yet know how salty or acidic Europa’s waters are, making it difficult to match the packaging with its eventual underwater environment. Her team will test sensors in frigid Alaskan waters and other areas around the world.
Closer to home, and someplace a lot warmer, Chris Carr is on the science team that will explore the clouds of Venus in 2026. The AE assistant professor and his students are working on the Venus Life Finder mission with MIT and a company called Rocket Lab. They’ll send a probe to Earth’s “evil twin” to search for signs of organic chemistry in its atmosphere. It will be the first private spacecraft to reach Venus, and the first U.S. mission to study its sulfuric acid-filled clouds in nearly 50 years.
Carr’s team helped oversee the development of the spacecraft’s autofluorescence nephelometer (AFN), which was built by Droplet Measurement Technologies. As it races through the Venusian atmosphere, the instrument will fire a laser through the clouds and use light scattering to measure the size and composition of the planet’s aerosols. The mission will also measure fluorescence, which Carr called a smoking gun for possible organic materials.
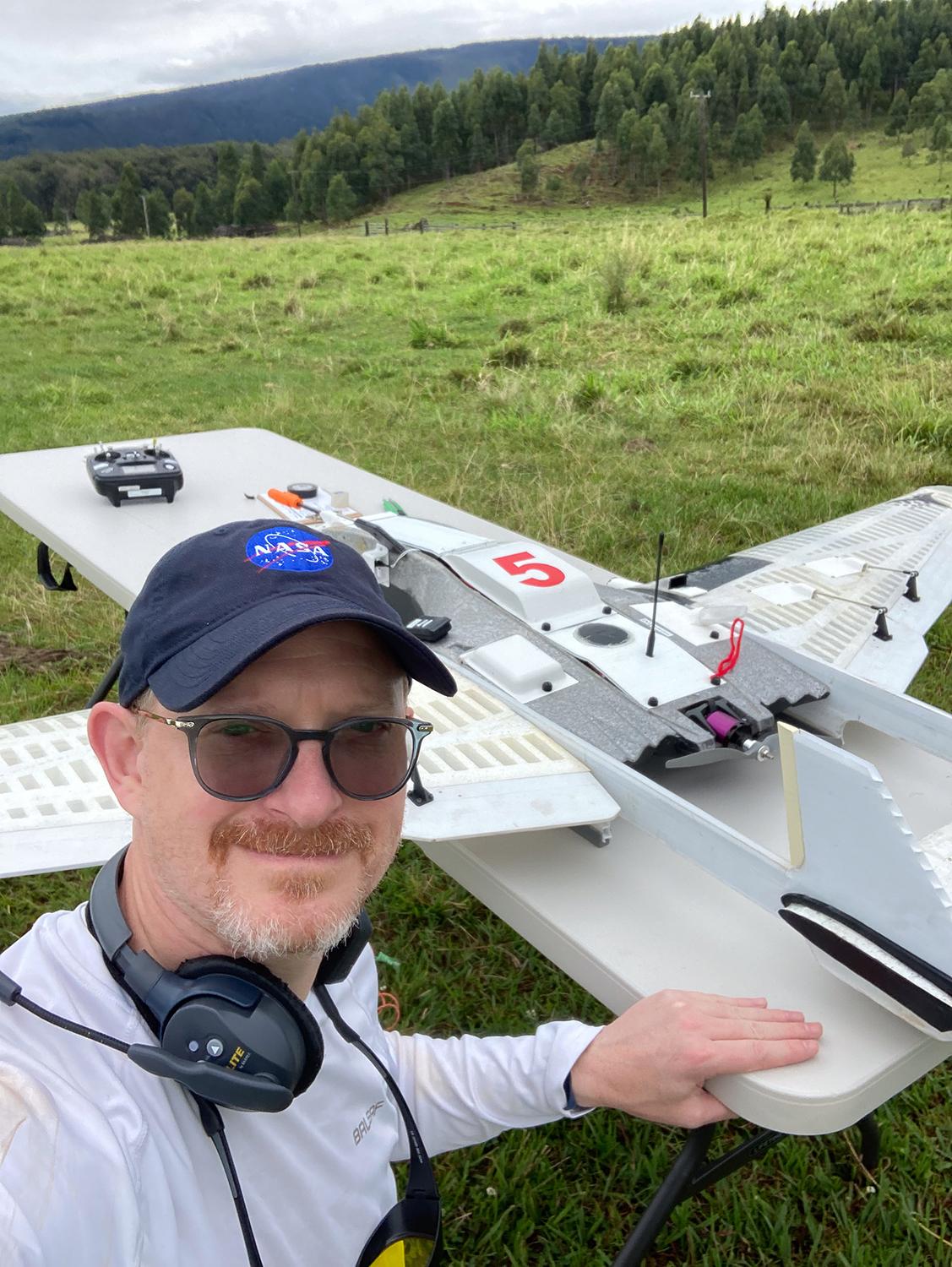
Chris Carr and his team flew a test craft through Hawaii’s volcanic fog clouds to gather baseline data for a 2026 mission to Venus.
“While I don’t think there is life as we know it in those clouds, it’s important to better understand Venus’ chemistry,” said Carr, who has a joint appointment in the College of Sciences’ School of Earth and Atmospheric Sciences. “Sulfuric acid and water have different properties, which can contribute to or limit the kind of chemistry that can occur. By understanding what might be possible, we can learn if different types of life might be possible. It also helps us know what to look for when we look for life.”
Carr’s team tested the AFN in March, flying it through Hawaii’s volcanic fog clouds, which are rich with sulfuric acid droplets. The measurements will serve as an important baseline to compare against what will be gathered on Venus. The probe will collect data for just five or six minutes and continue transmitting data to Earth for another 10-15 minutes before it exceeds its design limits and disintegrates. All after a journey that will take three to four months.
Carr’s work extends to our other planetary neighbor, too. He’s serving on a National Academies committee outlining the science priorities for the first crewed missions to Mars. He’s also sent several experiments to the International Space Station to learn more about how bacteria grow so that scientists can better fight antibiotic-resistant bacteria on long-duration space missions.
Crewed deep-space flights also are the focus for the AE School’s Álvaro Romero-Calvo. He’s working with NASA to find better ways to make oxygen for astronauts. Current systems aboard the International Space Station use electrolysis to separate water into hydrogen and oxygen. The machines are bulky with many moving parts. Backup equipment is stored on the station in case repairs are needed. And should a critical emergency occur, the crew could quickly return to Earth.
None of this is practical for an isolated astronaut crew millions of miles into their journey to or from Mars. Their smaller spacecraft will need simple, reliable systems. Carrying spare parts means more mass, and flying with more mass is more expensive. A quick return home in an emergency would be impossible.
Romero-Calvo is working with NASA on an oxygen system with no moving parts. He’s also splitting water into hydrogen and oxygen in microgravity using electrolysis. But Romero-Calvo then uses magnets to extract the oxygen, creating a process that avoids the need for a forced water recirculation loop, pumps, and centrifuges.
Mars-bound astronauts breathing Romero-Calvo’s magnet-made oxygen. Cressler’s transistors reaching Europa. Ansari’s sensors swimming in an ocean 400 million miles away. They’re all projects and missions that might not be a reality for at least a decade. But preparing for the future is what excites Ansari the most.
“Sure, our projects are a little blue sky. I know they won’t happen soon,” she said. “But it’s good to think about ideas that are far away. That’s the role of academia — to think ahead to projects, ideas, and solutions that will be deployed many years into the future.”
(text and background only visible when logged in)
Space, planet, and moon images courtesy: NASA / JPL
(text and background only visible when logged in)
Related Stories
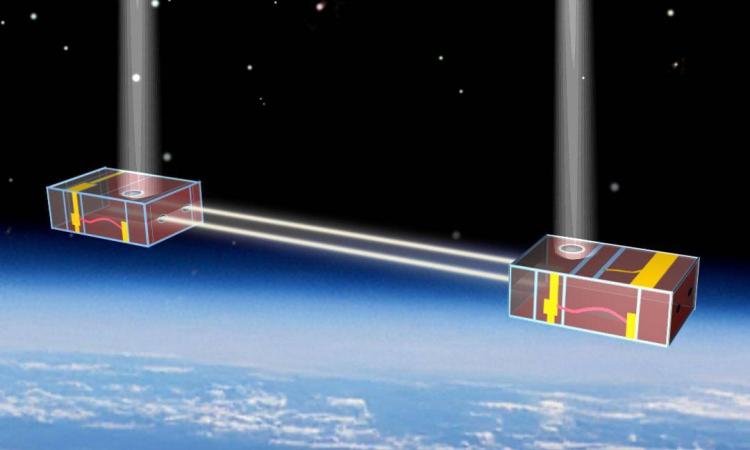
Turning to CubeSats in the Search for Life Thousands of Light-Years from Earth
Georgia Tech plays a starring role in NASA’s STARI mission to determine if telescope technology that studies exoplanets can be implemented in briefcase-sized spacecraft.
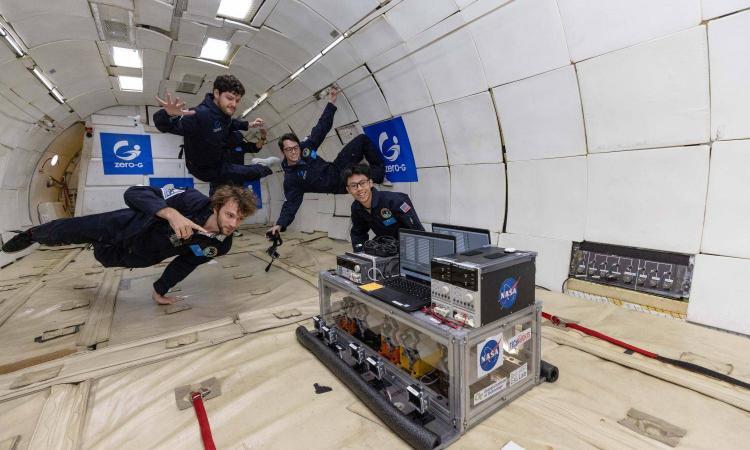
CubeSat Propellant Innovation Set to Transform Space Missions
Four aerospace researchers rode aboard a plane in zero-gravity conditions to test payloads and multiphase fluid management technologies.
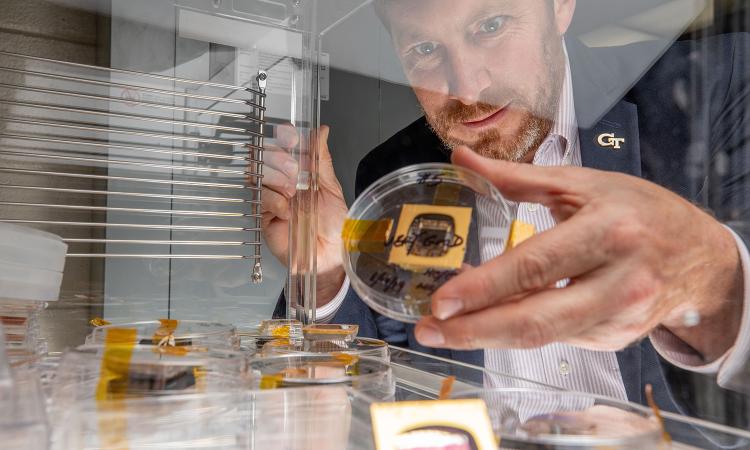
10 Questions with Jud Ready
Space researcher. Materials scientist. Entrepreneur. And Yellow Jacket.
(text and background only visible when logged in)
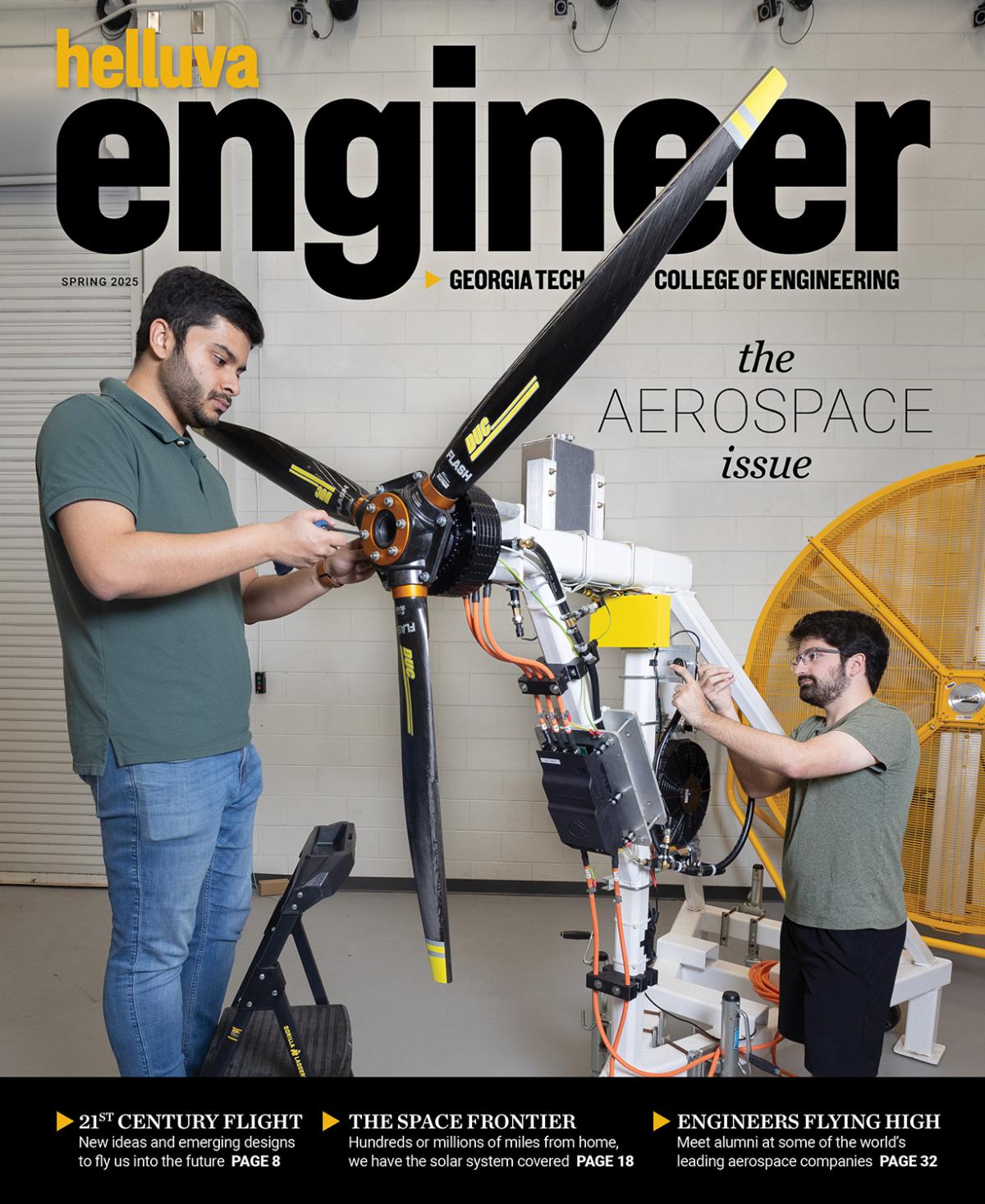
Helluva Engineer
This story originally appeared in the Spring 2025 issue of Helluva Engineer magazine.
Not where we are, but where we’re going — whether it’s here on the ground or millions of miles away. Georgia Tech engineers are shaping the rapidly shifting future of how we’ll fly people and stuff. Our engineers are helping get humans to the moon and, eventually, Mars, while creating the tools to help us unravel some of our solar system’s deepest mysteries. The sky is no limit in the Georgia Tech College of Engineering. Take off with us into The Aerospace Issue.