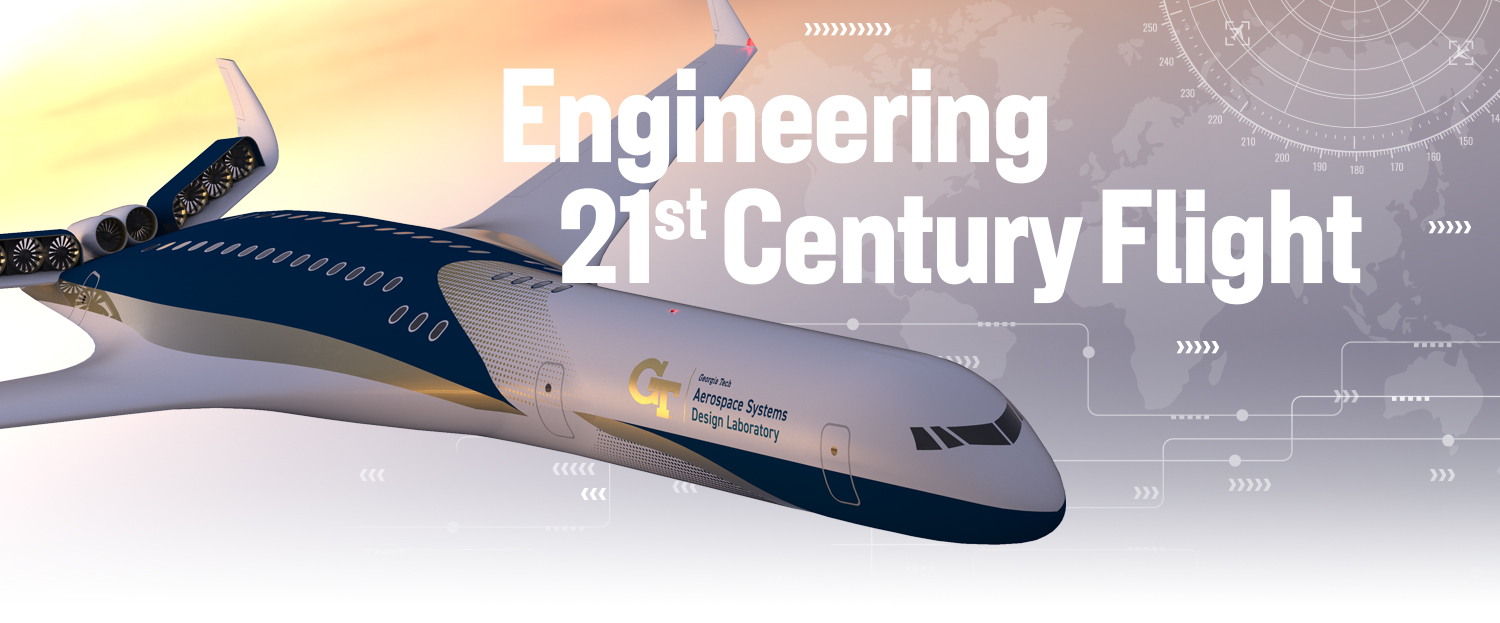
The new ideas and emerging designs that will carry us (and our cargo) safely into the sky will be more sustainable environmentally and economically thanks to the work of Georgia Tech engineers.
(text and background only visible when logged in)
When a military helicopter and a passenger jet fatally collided over the Potomac River in Washington D.C. earlier this year, the tragedy illustrated a stark truth: Our skies are crowded. And they’re only getting more so.
When the international trade group for airlines committed in 2021 to “fly net-zero by 2050,” the pledge underlined a growing problem: Aviation already accounts for 3% of global carbon emissions. That’s going to balloon if passenger miles double by 2050, as expected.
When journalists produce a steady stream of headlines about autonomous air taxis, the implication is clear: New electrically powered vertical takeoff and landing (eVTOL) aircraft suggest flying could look wildly different in some corners.
On the front lines of each of those issues — and more — you’ll find Georgia Tech engineers doing the critical research to smooth out the turbulence of a dynamic and changing industry. From designs that move beyond the tube-and-wing flying the skies today to new ways to model passenger demand and offer new kinds of travel packages, Yellow Jacket fingerprints are everywhere.
“Many of these things that are happening [in aviation] have roots directly here,” said aerospace engineer and Regents’ Professor Dimitri Mavris.
So grab a boarding pass, adjust your seat back, and see how engineers are planning, modeling, designing, and building the future of flight.
(text and background only visible when logged in)
(text and background only visible when logged in)
Meet the RAVEN
In an ordinary-looking hangar a few miles northwest of campus, the bones of a new kind of aircraft are taking shape. The steel frame won’t ever get seats or an aluminum skin — or actually fly, for that matter. But it’s a key stage in building a flying laboratory that will help NASA and Georgia Tech engineers research and test the technologies needed to develop eVTOL aircraft.
Eventually, the finished vehicle — called RAVEN — will look similar to the small general aviation planes common at executive and regional airports. But it will be entirely electric, autonomously piloted, and capable of helicopter-like vertical flight and hover with plane-like forward flight.
To speed development and keep costs low, the aircraft is based on the Bede BD-6, an experimental fixed-wing plane commercially available as a home-built kit. It will have four tilting rotors able to pivot from vertical lift and hovering to forward movement plus two additional static rotors to aid in vertical flight. Each of those six-foot-diameter rotors will be powered by its own 40-kilowatt electric motor — equivalent to about 50 horsepower each.
At 1,000 pounds, the RAVEN — Research Aircraft for eVTOL Enabling Technologies — is about 1/5 the size of the kinds of autonomous vehicles that could someday fly people and cargo. The large size is perfect to offer real insight into systems and designs for those future aircraft.
“At this weight, you’re a lot more relevant than a typical 20- or 50-pound research aircraft. The physics don’t scale in the same way. You need to be closer in size to the larger aircraft to get relevant results,” said Brian German, professor in the Daniel Guggenheim School of Aerospace Engineering (AE). “We want to be able to publish flight data that’s relevant and carry relevant research payloads to collect information about the aerodynamics, acoustics, electric propulsion system, and more.”
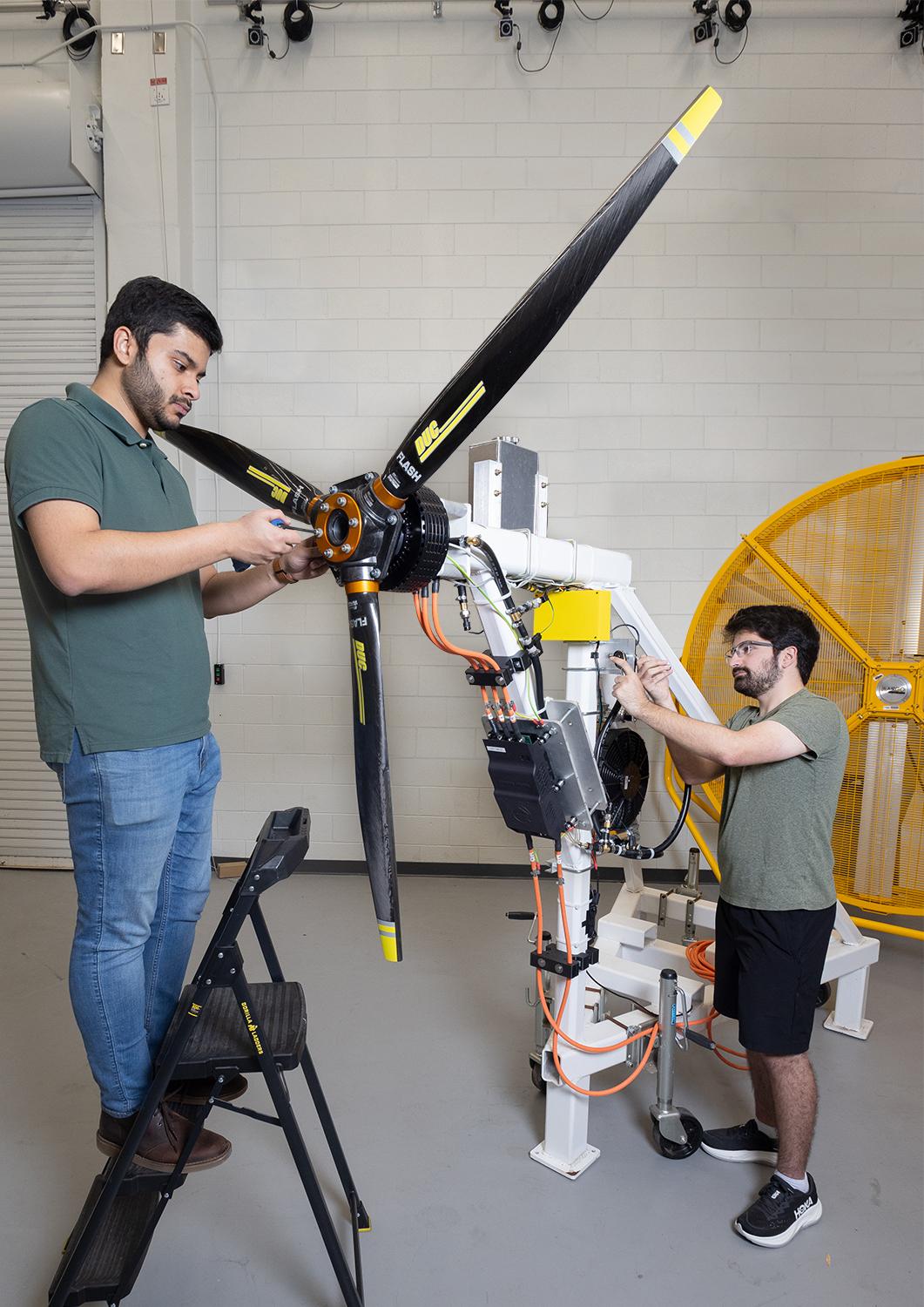
Maxwell Kramer, a master’s student in Brian German’s lab, and research engineer Ayusha Jha work on one of the RAVEN’s electric motors and a propeller ahead of ground tests. (Photo: Candler Hobbs)
German approached NASA a few years ago with the idea of building this kind of dedicated research platform at Tech that also would give students hands-on experience with experimental aircraft design and flight. The first steps of that journey will travel just a few hundred feet this summer, when German and his team roll the steel frame “iron bird” out to a closed runway to test everything. With cable runs, electric motors, and propellers laid out just like the airplane, it’s an important milestone to eventually realizing the actual RAVEN.
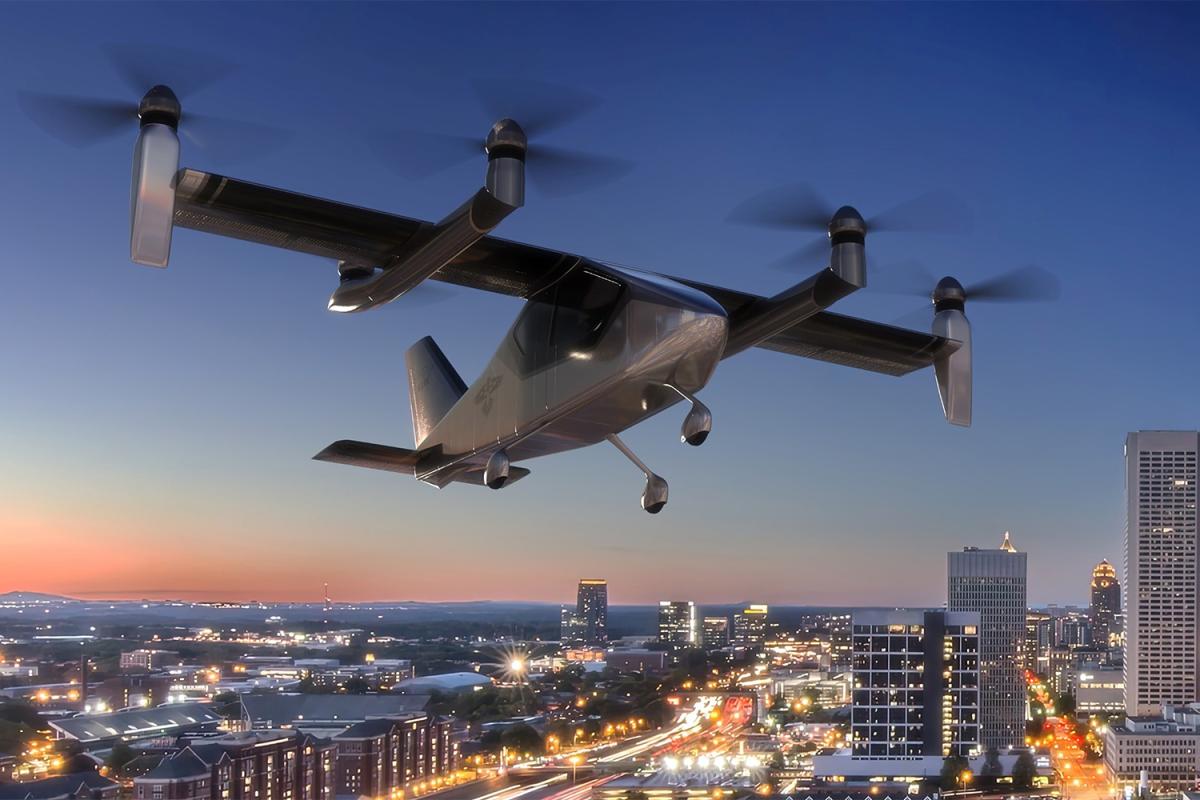
A conceptual rendering of the RAVEN in flight over Atlanta, Georgia.

Applying a rust remover to prepare the RAVEN iron bird for painting. (Photo: Candler Hobbs)
ADVANCED AIR MOBILITY
The phrase refers to a range of emerging aviation technologies and new aircraft designs to move people and goods. It’s an umbrella term that can include autonomous or human-piloted eVTOL craft used for short trips, drones, and more. In urban areas, these kinds of innovations can be more specifically called “urban air mobility” or “regional air mobility.”
“We’ll run it up and take a lot of data,” German said. “Can we produce the power and thrust levels we needed? Do we have any electromagnetic interference? That’s a big deal. We have a high-voltage, high-power electrical system — does that interfere with the low-voltage command and control signals that control everything? Do any of the components overheat? Those are the kinds of things we’ll be looking at.”
Meanwhile, a new hangar facility rising in Georgia Tech’s North Avenue Research Area will offer new space for testing and development of the RAVEN’s systems. The Aircraft Prototyping Laboratory (APL) also will house an electric powertrain lab, a propulsion system test cell, an avionics lab, and composite fabrication areas. Scheduled to open in fall 2025, the APL will complement the off-campus airport hangar where the RAVEN’s iron bird is coming together, German said.
“My vision is for Georgia Tech to have an ecosystem around research and flight testing for advanced air mobility,” he said. “The end state is flight testing, but the other aspects are also super interesting — our graduates will have a rich set of experiences that make them even more valuable to the industry and our work will become a major enabler of bringing businesses and an advanced air mobility workforce to Georgia.”
(text and background only visible when logged in)
(text and background only visible when logged in)
Windy Cities
If eVTOL vehicles are coming to skies near you, there’s much to do beyond designing the vehicles themselves. A signature research effort looking at the broader picture has been housed at Georgia Tech for more than 40 years.
The Vertical Lift Research Center of Excellence focuses on all areas of vertical lift: traditional helicopters and other rotorcraft, urban air mobility vehicles and drones, and even rotorcraft that could one day help us explore other planets and moons.
Led by Marilyn Smith, the Center operates on a five-year cycle with funding from NASA, the U.S. Army, and the U.S. Navy. Three years into the current cycle, $16 million worth of research is underway on a dozen projects involving 10 faculty members and six universities. The Center also has become a hub for training — one of the few places in the country with a full scope of curriculum for rotorcraft engineers.
Smith is a respected scholar in aerodynamics and aeroelasticity, studying the interactions of air and aircraft structures. And for a long time, she’s pushed for more study of those interactions in the congested urban areas where advanced air mobility flights would operate.
“When advanced air mobility vehicles are performing terminal area operations — taking off and landing — they will not be above the building line where we typically fly aircraft. You’re not in a nice, open airport area,” said Smith, the David S. Lewis Professor in AE. “A significant issue is that the vehicles will encounter many transients with the wind: There’s a lot of turbulence as the wind moves around buildings and other structures, and there will be vortices that you have to fly through. That’s a considerable safety issue, especially during takeoff or landing.”
Smith said pilots and aircraft will need to be able to predict and adapt to these conditions. That means designing aircraft for a significantly different flying environment and training pilots for the conditions. It also means establishing operational parameters — Can we fly in today’s conditions? What routes and trajectories are safest?
To that end, Smith and her team have developed tools and models for the last few years that would predict conditions where nonlinear flight would occur, particularly in what’s known as the “roughness sublayer” of air — building heights plus 50%. Smith is working with a company that develops industry-standard models and simulations to incorporate her group’s work into flight simulators.
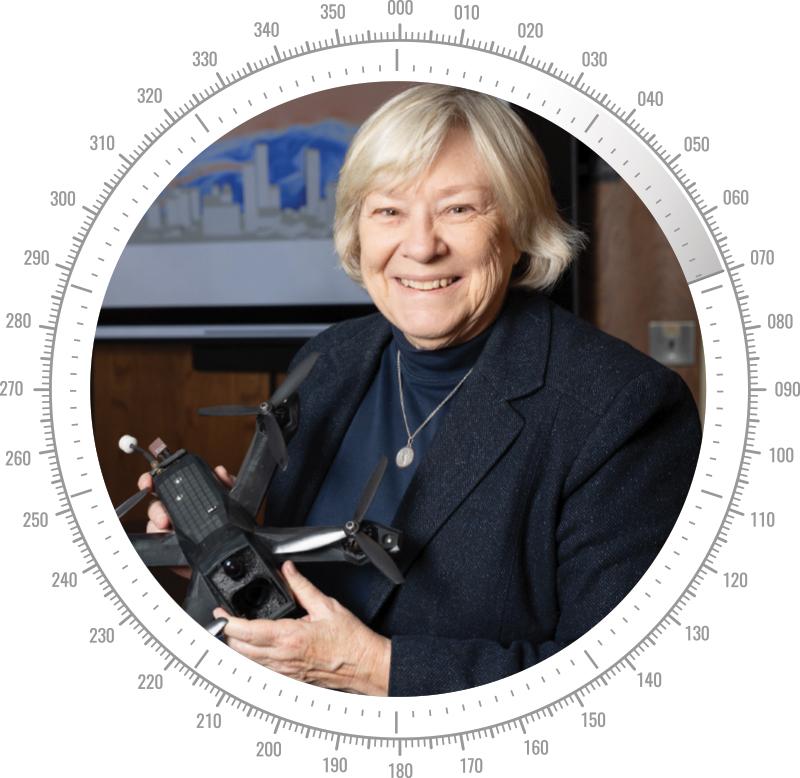
Marilyn Smith (Photo: Candler Hobbs)
It’s potentially life-and-death work if air taxis and drones are to become viable operators.
“Are you designing the vehicle so that it can be the most productive flying through these areas? You don’t want conditions to draw too heavily on the battery so that your battery drains too fast,” Smith said. “If you’re flying a lighter drone, can you actually fly it on that path that day, or is it going to get slammed into the side of a building because it’s just too windy?”
A significant issue is that the vehicles will encounter many transients with the wind: There’s a lot of turbulence as the wind moves around buildings and other structures, and there will be vortices that you have to fly through. That’s a considerable safety issue, especially during takeoff or landing.
MARILYN SMITH
In a related project for NASA — especially timely after this winter’s raging wildfires in California — Smith is modeling the environment around wildfires and fires in urban areas. Her models predict the direction of spread and thermals generated by the intense heat to offer insights on potential impacts and ideal flight paths for drones dropping fire retardants.
Another area of research in Smith’s lab looks at what happens when tilt-rotor aircraft like the RAVEN transition from hover to forward flight.
“We don’t have good databases for solver validation and to understand the physics for tilt-rotor conversions,” Smith said. “[AE Assistant Professor] Juergen Rauleder is performing extensive experiments on that topic, and we are studying the aerodynamic interactions with him. We’re also making sure we can capture the important physics with the simulation tools we have and develop best practices to capture the physics.”
Smith said the world of advanced air mobility has shifted from feasibility to the just-as-complex questions of operations, which is why her group’s work is crucial.
“People weren’t thinking about this before, because they were focused on so many other things: Can you build vehicles? Are they practical?” she said. “At this point we’re saying, ‘We can build vehicles. They’re practical. Now, how do we operate them safely? What are these other problems that we need to solve?’”
(text and background only visible when logged in)
(text and background only visible when logged in)
The View from 30,000 Feet
The question of safe and efficient operations for advanced air mobility services like cargo flights or air taxis is one of the topics that occupies attention in the Aerospace Systems Design Lab (ASDL). The lab works on advances in civil aeronautics, challenges in air transportation, environmental assessments, and guiding international aviation organizations in setting long-term goals for the industry.
It’s a holistic approach to complex aerospace systems, said ASDL Director Dimitri Mavris. That’s partly why the lab’s fingerprints, and Georgia Tech’s, can be found across many of the ambitious efforts to reimagine aerospace designs. For example:
- NASA’s X-59 aircraft designed to demonstrate supersonic flight with a sonic “thump” rather than a sonic boom. The project is assessing ground-level noise in an effort to allow for faster-than-sound flight over land. One of Mavris’ former Ph.D. students, Michael Buonanno, was the project’s chief engineer. He’s currently a Lockheed Martin Fellow.
- Another former ASDL student, Nicholas Borer, now at NASA’s Langley Research Center, was deeply involved in developing the X-57 experimental aircraft “Maxwell.” The plane’s distributed electric propulsion design was to have 14 electric motors and propellers along its wings. Though it never expanded beyond ground testing before running out of time and money, the project resulted in significant advances in batteries and other electric aircraft technology.
- Working with Virginia Tech, the lab established the fundamental analysis of the truss-braced wing concept. The work became the springboard for the X-66, another NASA experimental plane that’s focused on one day achieving net-zero aviation emissions. The extra-long, thin wings of the design are stabilized by diagonal struts. Combined with advances in propulsion, materials, and systems architecture innovations, the X-66 could use 30% less fuel and produce fewer emissions than today’s best aircraft.
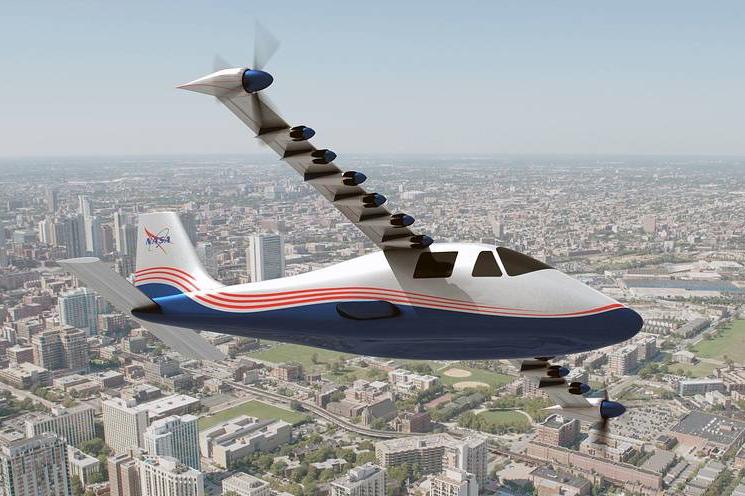
NASA’s X-57 Maxwell
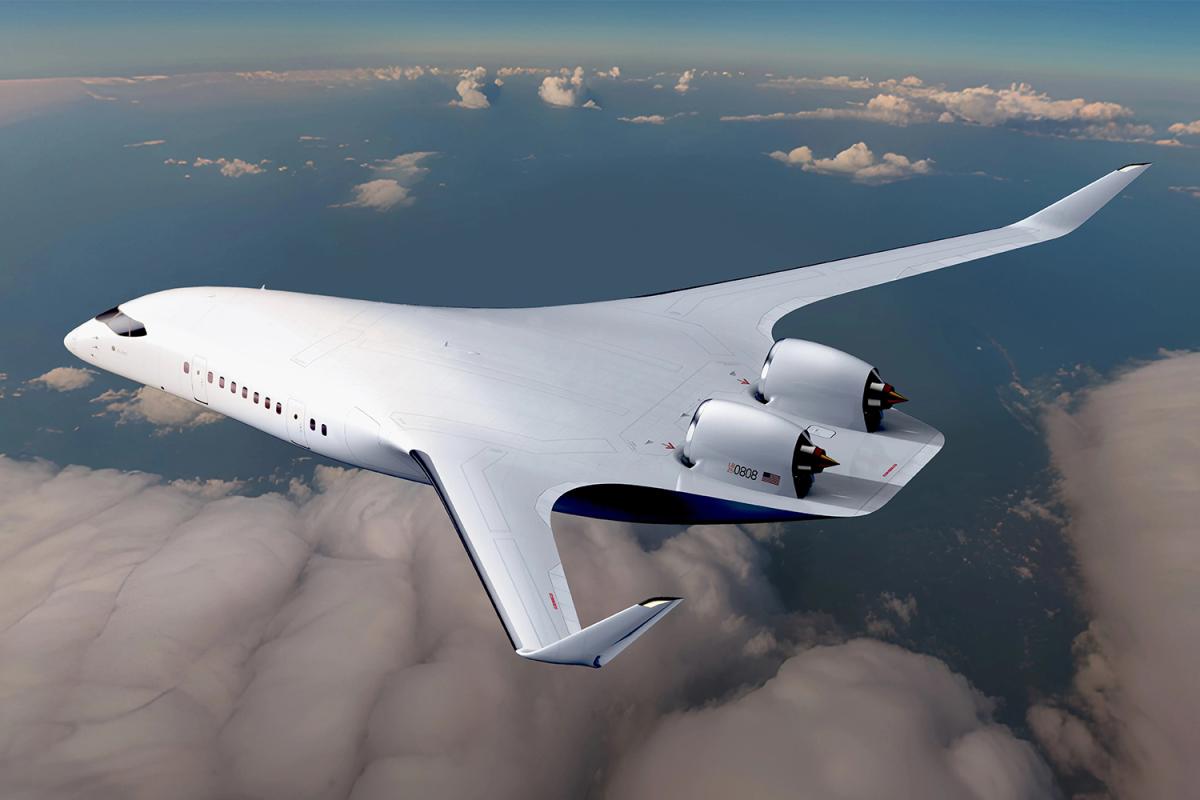
NASA and Jet Zero’s blended wing aircraft
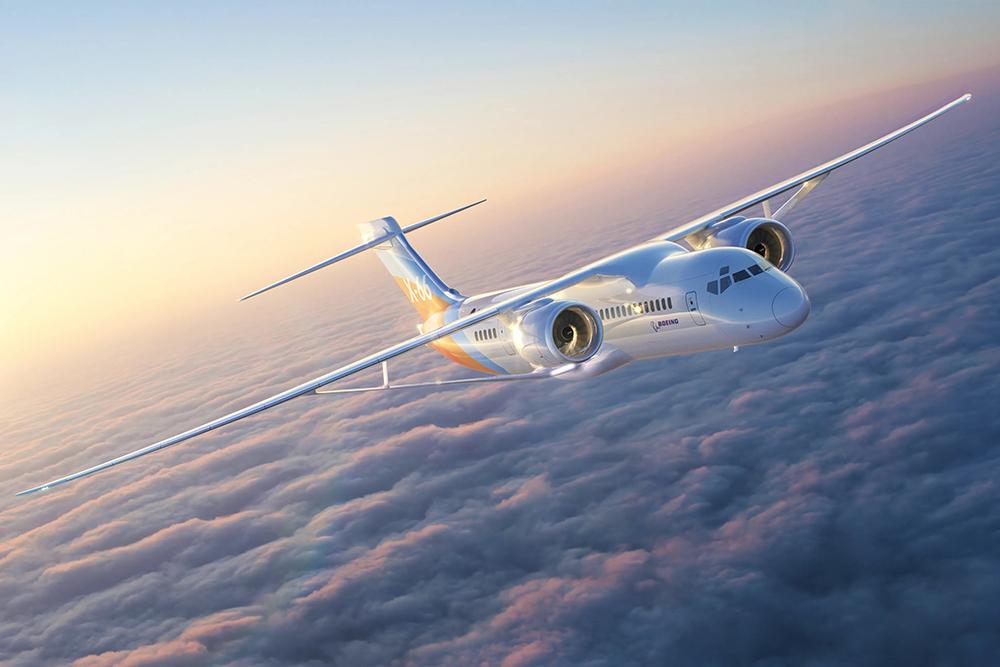
NASA’s X-66 experimental aircraft
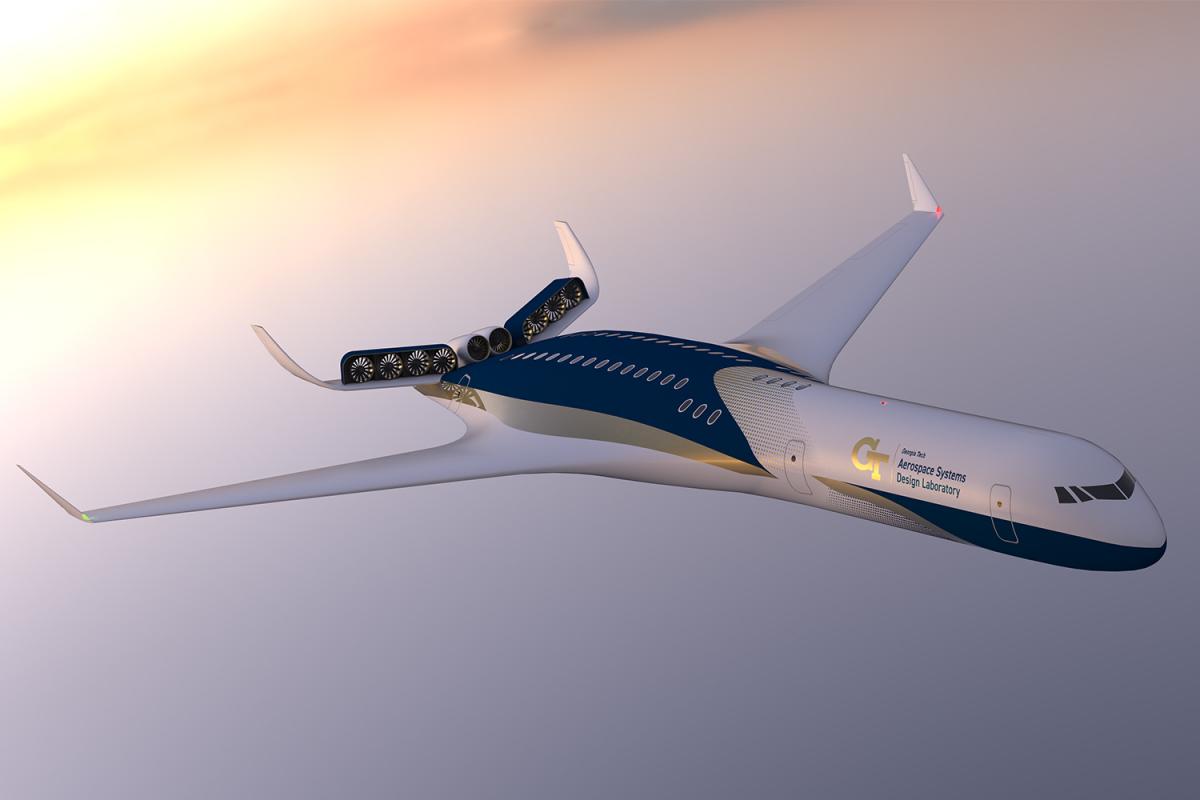
NASA’s ATH2ENA aircraft
“For many of these configurations, there is a bit of quote-unquote Georgia Tech heritage, if you will, from our lab and from the Guggenheim School of Aerospace Engineering,” said Mavris, who is the Boeing Professor of Advanced Aerospace Systems Analysis, Langley Distinguished Professor in Advanced Aerospace Systems Architecture, and Distinguished Regents’ Professor in AE.
Mavris’ team has been involved in evaluations of another new approach called a blended wing body design with a company called JetZero. With a shorter, wider fuselage that blends into a wing, it’s a vastly different profile than traditional tube-and-wing aircraft. But it’s a more naturally stable design that’s lighter and has less drag, boosting efficiency while eliminating the need for large and complex tail surfaces.
Echoes of the blended wing body show up in one of the lab’s newest projects through the NASA Advanced Aircraft Concepts for Environmental Sustainability 2050 program. Called ATH2ENA, the design merges tube-and-wing design with a blended wing in a concept for a future airliner.
The ATH2ENA idea uses a hydrogen-electric propulsion system, which is where it gets its full name: Advanced Technology Hydrogen Electric Novel Aircraft. Its unique shape produces a higher lift-to-drag ratio that makes it more efficient while maximizing cryogenic hydrogen storage and balancing weight.
“In a typical tube-and-wing, the passenger cabin is constrained by placement of the fuel tanks — passengers can feel like they’re going through the tank. In this case, the cabin is in the middle of that blended body and the tanks are not on top of the passengers. So, it has some appeal,” Mavris said.
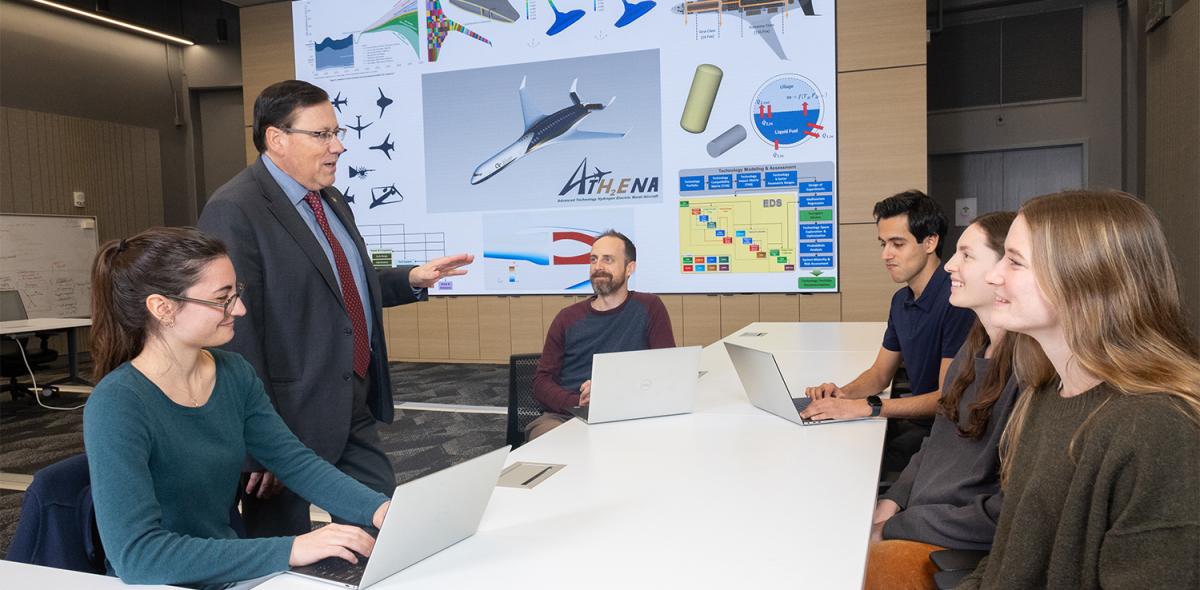
Dimitri Mavris discusses ATH2ENA with ASDL researchers and students. From left: Ariadne Papamichou, Mavris, Christian Perron, Jai Ahuja, Brinley Rawson, and Sarah Treece. (Photo: Candler Hobbs)
The ASDL team was the only university-based group selected for the research program. ATH2ENA is really the endpoint of their work. Researchers will develop a comprehensive assessment of sustainable technologies — alternative fuels, propulsion systems, and aircraft designs. The most promising approaches will inform the ultimate ATH2ENA design and development.
And that gets back to the 30,000-foot view ASDL has over aerospace systems. Yes, their work explores new technology for vehicles. But more and more, the team looks closely at how all the infrastructure, operations, and new vehicles come together for a more sustainable flying future.
“We are the ones architecting what the system should look like and what breakthroughs it will take to get there,” Mavris said.
Consider the matrix of issues with new types of fuel or propulsion systems.
How would new kinds of sustainable aviation fuels be transported to an airport such as Hartsfield-Jackson Atlanta International Airport? New pipelines would have to be built, or new nearby facilities established so fuel could be trucked in.
I think we’re in a very unique nexus of capabilities and size. We have enough volume and resources and credibility — and that also helps in attracting the very best students in the domain.
DIMITRI MAVRIS
Current restrictions on hydrogen means aircraft refueling would have to happen at least 75 feet away from the terminal. Do planes move for refueling? Do passengers board buses to get to their plane on the tarmac?
Electric propulsion isn’t yet powerful enough for commercial jetliners, but ground equipment could be electrified (and in fact airlines are working on just that). But then airports need charging infrastructure. And that’s aside from the resources needed to offer charging for personal vehicles and rental car fleets.
And if an ATH2ENA-like design does become the future of passenger travel, adjustments will certainly be needed at airport terminals to accommodate something other than tube-and-wing planes.
They’re all big questions. And they’re all challenges Mavris and his team of 50 researchers and 300 graduate students are fully engaged in.
“The field is pretty vast, you know. I think we’re in a very unique nexus of capabilities and size,” Mavris said. “We have enough volume and resources and credibility — and that also helps in attracting the very best students in the domain.”
(text and background only visible when logged in)
(text and background only visible when logged in)
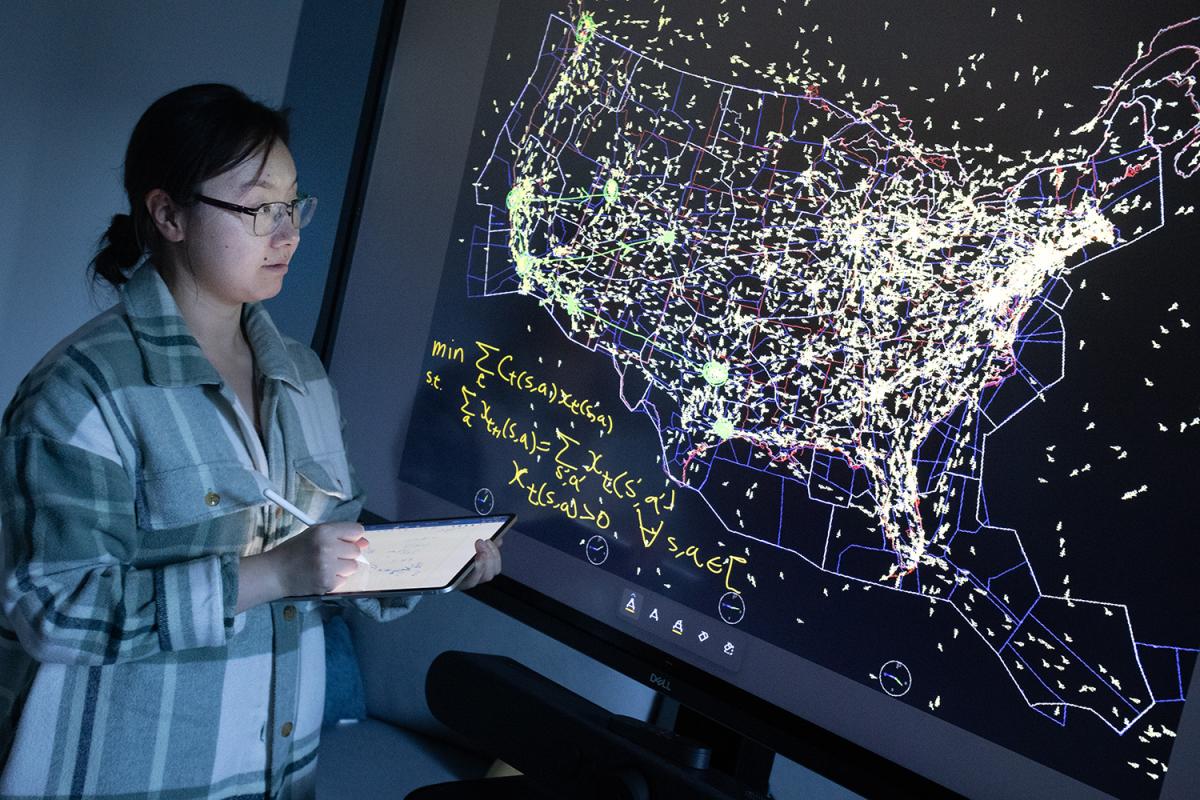
Sarah Li with a map of a typical day’s air traffic. (Photo: Candler Hobbs)
Gaming Out Air Traffic
As Marilyn Smith noted, the complicated operations of autonomous air taxis or package delivery drones would add complexity to our airspace. And that’s atop the growing congestion from conventional air travel.
Newly arrived AE Assistant Professor Sarah Li is applying the principles of game theory to air traffic routing and management.
Typically, planning for flights happens a year or more out, allowing for route and timing adjustments to avoid conflicts. Then during actual flight operations, air traffic controllers monitor a portion of the sky to ensure aircraft avoid each other and make it safely to their destinations. As new kinds of vehicles traveling shorter routes in more confined spaces start to contribute to that mix, it’s going to be too much for the current system to keep track of.
“Traditionally, maybe it didn’t make as much sense to apply game theory here, but now I think it will,” Li said. “We’re losing some of that centralized structure. Imagine you order something from Amazon in the morning — and let’s say advanced air mobility happens. Amazon is going to ship it out in the afternoon. You maybe get a six-hour window to insert that flight trajectory into the current system. We can’t manage air traffic the same way we do now, where we have two years’ lead time to deconflict everything.”
Li sees her work augmenting human controllers trying to process and account for all the constantly updating information available to them. Her models might pull in all that data and suggest optimal routes to avoid conflicts or circumvent developing weather issues. This kind of partnership could mean better overall air traffic handling.
“Controllers have some idea of what’s going on broadly, but they are mostly focused on local traffic control. Let’s say there are three or four different routing options that they’re more or less OK with locally. Maybe our models could suggest, based on global weather information or global forecasted traffic, you should choose this one if you can,” Li said.
To oversimplify, Li’s models have three main parts. First, they deal with multi-agent systems — in other words, all the individual vehicles using airspace at the same time — and the constraints and objectives for each of those agents/aircraft. Those objectives might be to arrive at their destination on time and use the least amount of fuel.
Second, the model accounts for the system’s dynamics: Behavior and activity change over time, and they happen in sequence. And third, the model incorporates uncertainty: weather forecasts, miscommunications, delays on the ground, and more.
What makes game theory an interesting approach, Li said, is that the objectives of any one flight might be in conflict — or at least affect — other flights. Every “agent” in the system can’t achieve its on-time, fuel-efficient objective without routes that would cause collisions or at least very close calls.
“We want to optimize the objective given constraints,” Li said. “Now these objectives and constraints are coupled to another optimization problem, they’re coupled with respect to each other. That’s game theory.”
She said it’s a valuable approach for thinking about managing the realities of ever-busier skies.
“Game theory is a good framework where now you can look at these multi-agent systems and how their decisions affect each other.”
(text and background only visible when logged in)
(text and background only visible when logged in)
Predicting Flutter to Prevent It
AE Assistant Professor Cristina Riso is using computational methods to predict and understand how new kinds of aircraft — such as eVTOLs — will vibrate in flight as a result of their interactions with the surrounding airflow.
Riso’s work focuses on aeroelastic instabilities that aircraft wings, tilting rotors and propellers, and other aerospace structures might encounter aloft. It’s perfectly normal for these structures to vibrate in flight due to turbulence or wind gusts. Typically, the vibrations end fairly quickly.
However, when they continue to grow in amplitude, it’s called a flutter instability — and it’s a key safety consideration in developing aircraft. Riso is working on methods to predict, understand, and prevent flutter early in the design phase. The methods also may be useful for other structures exposed to fluid flows, including large-scale wind turbines or even rotorcraft used to explore other planets.
“We are interested in developing methods that can be used across the spectrum of aerospace,” Riso said. “That could be an existing conventional aircraft that we try to make lighter-weight or more aerodynamically efficient, which requires us to understand how design changes impact in-flight vibrations. But also, we want to understand how to design new configurations.”
Engineers know a lot about flutter for conventional airplanes and helicopters, but they don’t have the same mature knowledge for emerging designs. For example, aeroelastic instabilities such as flutter can be intensified in proposed aircraft designs with lighter-weight, longer wings and in completely new configurations for air taxis or cargo drones. Riso said it’s key to understand those issues early on and optimize designs from the get-go.
“If you find the problems later, such as when performing flight tests, then you have to alter your design in suboptimal ways and delay the development process,” Riso said. “By having systematic ways to characterize these instabilities and potentially consider them earlier in the design phase, we may achieve better performance than the current process produces while maintaining safety standards.”
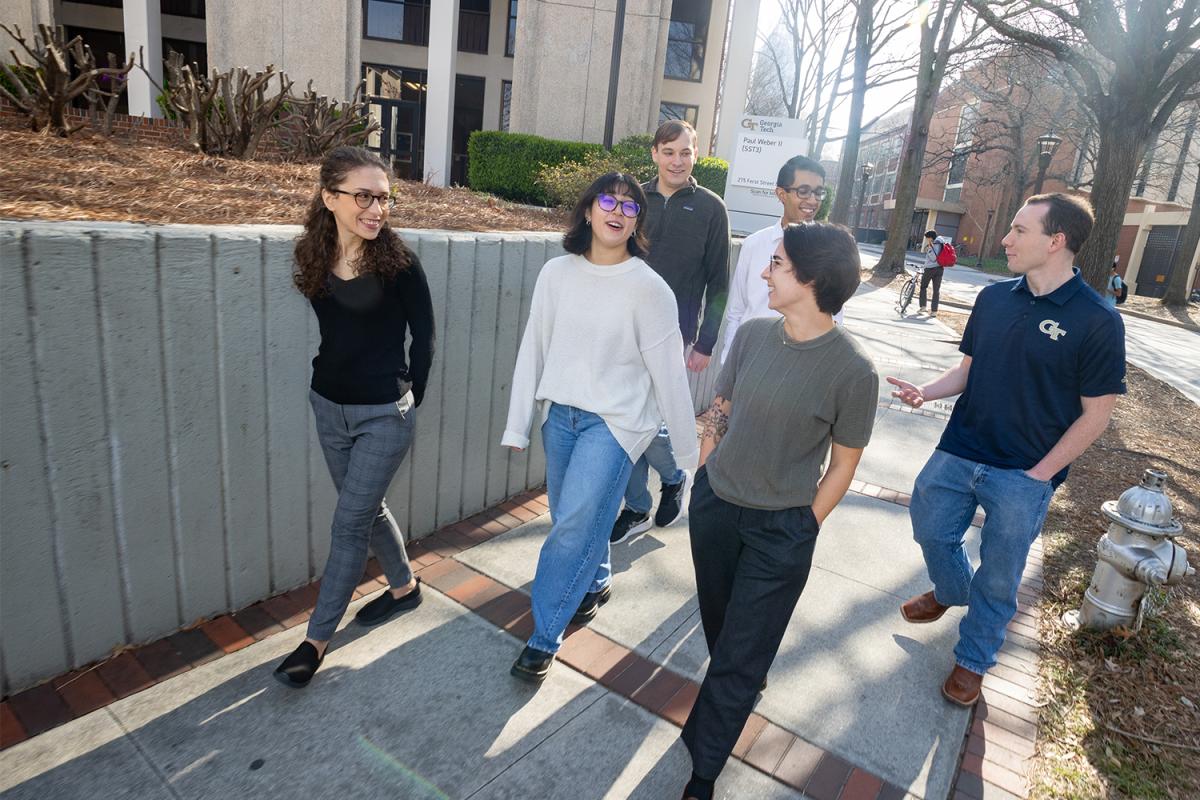
The Riso group discusses flutter. Front row, from left: Cristina Riso, Isabelle Sanz, and Maia Gatlin. Back row, from left: Gray Simmons, Amit Hegde, and Michael Hartim. (Photo: Candler Hobbs)
What was, at some point, a solved problem in the aerospace field has become a significant design challenge and highly active research field again, thanks to the push for new aircraft designs.
CRISTINA RISO
Some of the projects in Riso’s Structural Dynamics and Aeroelasticity Research Lab are focused on predicting instabilities for tilt-rotor and wing-propeller designs akin to what German and his team are exploring for the RAVEN.
Engineers encountered flutter instabilities in the 1960s in aircraft with flexibly mounted engine-propeller combinations. Called whirl flutter, it resulted in several major crashes before new prediction methods and design guidelines solved the problem. Now it can be an issue again.
“What was, at some point, a solved problem in the aerospace field has become a significant design challenge and highly active research field again, thanks to the push for new aircraft designs for more sustainable air transportation and the emergence of tilt-rotor and multi-propeller concepts for advanced air mobility,” Riso said. “These configurations are designed to be lighter-weight and more aerodynamically efficient. That makes for a more flexible structure, which exacerbates aeroelastic instabilities.”
(text and background only visible when logged in)
(text and background only visible when logged in)
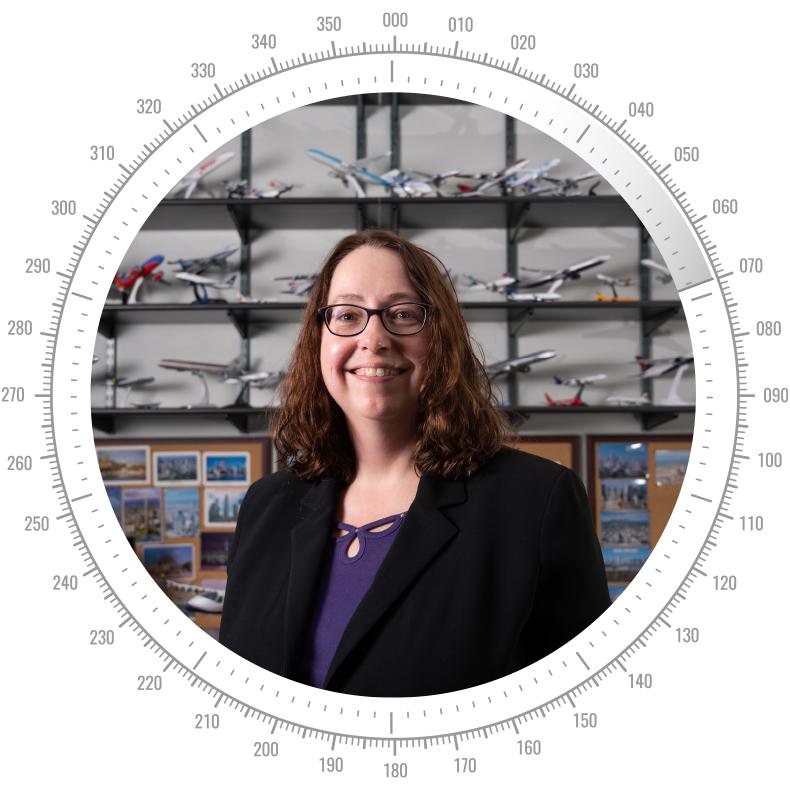
Laurie Garrow (Photo: Allison Carter)
Bundling Better Service for Passengers
After all this talk about new aircraft designs, air taxis, and managing air traffic, what about the traveling public?
In Laurie Garrow’s Air Transportation Lab at Georgia Tech (ATL@GT – get it?), researchers are working closely with airlines and travel technology companies to anticipate demand for air travel and develop new ways of packaging flights that benefit flyers and operators.
With airline and industry partners — among them United, Alaska, and Atlanta’s hometown airline Delta — the lab is developing next-generation models and simulations to balance customer demand and airline revenue.
“We are like the auditor. I’m not advocating for one particular revenue management system or one particular airline,” said Garrow, professor in the School of Civil and Environmental Engineering and AE. “Our role is to be neutral and guide development for the industry, to really evaluate pros and cons.”
The air travel industry is investing in new technology that will allow creation of customized bundles for individual consumers and allow consumers to compare similar offers across airlines. It’s a new, modern, and standardized communication protocol. And it will mean, for example, more tailored options for flyers and less significant price jumps between fare classes.
Travel packages could include a checked bag, advance seat assignments, or premium lounge access. But they also could include new kinds of nontraditional offerings — maybe free or discounted stopovers to break up long-haul flights. Garrow said the possibilities are vast. And the research need is helping airlines figure those out.
“We’re the math behind the scenes building a better airline experience, offering you products you actually want to buy and lower prices to consumers without steep price jumps you may see right now,” Garrow said. “We’re helping the airline industry stay profitable by finding good ideas and helping them better implement those ideas.”
The lab’s tools can help airlines decide what offers to create for customers, how to price them, and how to promote them to flyers. Their algorithms allow airlines to separate good ideas from bad ones by simulating the impact of those offers or other changes to service.
“Revenue management is such a strong lever. Small changes can have huge impacts on the bottom line or lead to huge losses,” Garrow said. “The value proposition we bring is this ability to model almost the full airline industry — competitive networks, competitive pricing, and revenue management — and allow researchers and industry partners to test out new ideas and quickly evaluate their impact.”
Garrow said her team’s work also helps airlines consider aircraft design questions and anticipate customer needs. They can help decisionmakers figure out when planes need more seats with extra legroom or more premium seats, for instance.
Garrow has long been a go-to resource for the industry. So, when the founder of a research consortium at MIT retired a few years ago, she worked quickly with eight airline and industry partners to establish ATL@GT. Along with research, those connections have also led her to create a graduate-level course on airline revenue management to fill a gap center members identified.
“No two companies are the same, so our role is coming up with a portfolio of the most important research questions that will benefit the industry and consumers.”
(text and background only visible when logged in)
(text and background only visible when logged in)
Related Stories
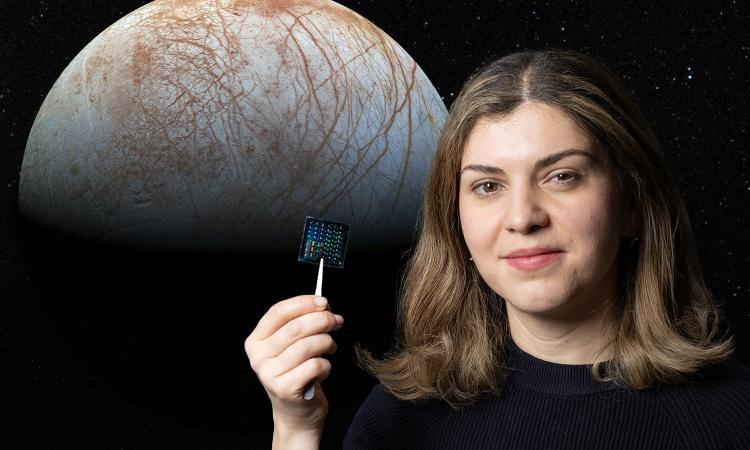
Space: The Current Frontier
Our engineers have the solar system covered, with projects hundreds — or millions — of miles from home.
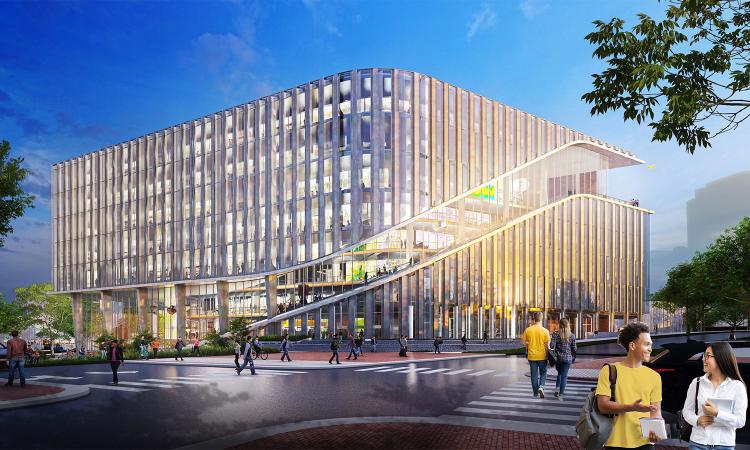
Building for the Future
A top program needs top facilities. Why it’s time for a new AE building to match the quality of the program’s education and research.
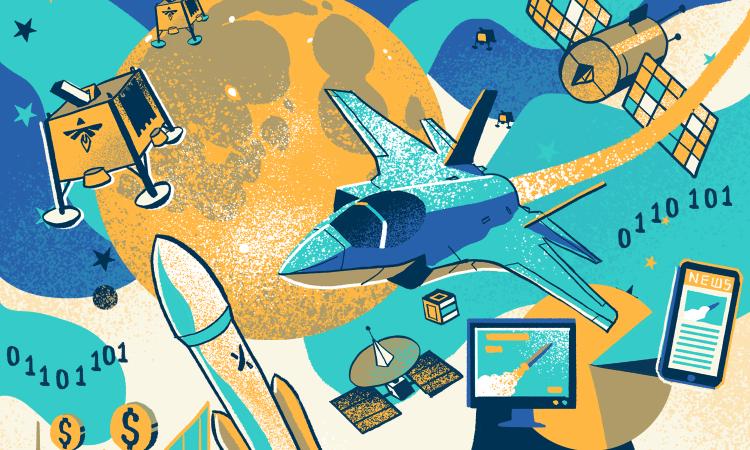
These Engineers are Flying High
Meet alumni working at some of the world’s leading aerospace organizations to innovate, fly safer, and travel farther.
(text and background only visible when logged in)
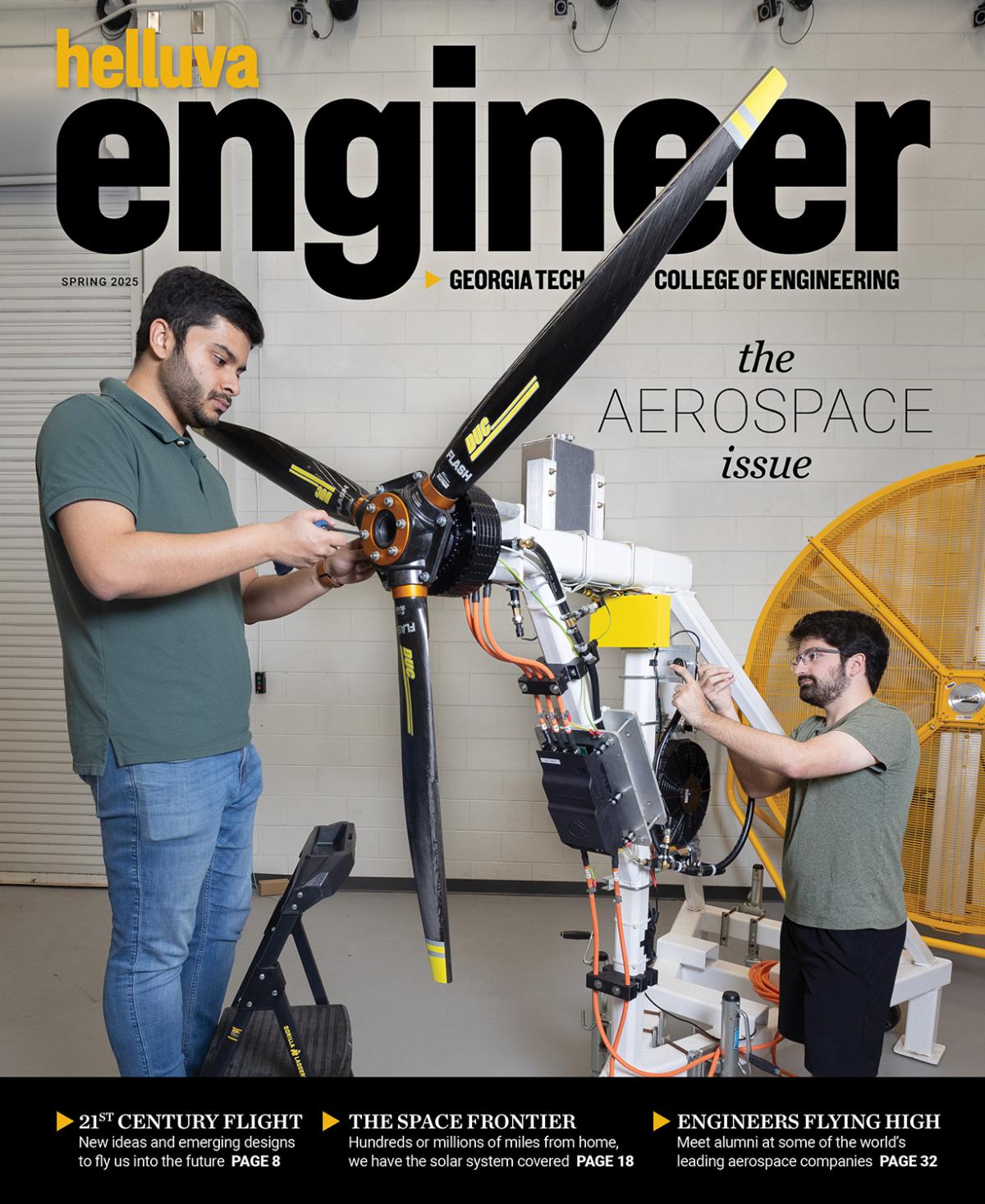
Helluva Engineer
This story originally appeared in the Spring 2025 issue of Helluva Engineer magazine.
Not where we are, but where we’re going — whether it’s here on the ground or millions of miles away. Georgia Tech engineers are shaping the rapidly shifting future of how we’ll fly people and stuff. Our engineers are helping get humans to the moon and, eventually, Mars, while creating the tools to help us unravel some of our solar system’s deepest mysteries. The sky is no limit in the Georgia Tech College of Engineering. Take off with us into The Aerospace Issue.